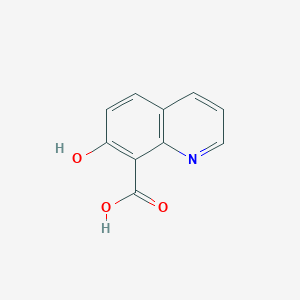
7-Hydroxyquinoline-8-carboxylic acid
Overview
Description
7-Hydroxyquinoline-8-carboxylic acid is a derivative of quinoline, a heterocyclic aromatic organic compound. This compound is known for its diverse biological activities and is widely used in various scientific research fields. The structure of this compound consists of a quinoline core with a hydroxyl group at the 7th position and a carboxylic acid group at the 8th position.
Mechanism of Action
Target of Action
7-Hydroxyquinoline-8-carboxylic acid, like other 8-Hydroxyquinoline derivatives, is considered a “privileged structure” that can bind to a diverse range of targets with high affinities . It has been found to interact with 2-Oxoglutarate (2OG) and iron-dependent oxygenases, which are considered promising therapeutic targets for various human diseases .
Mode of Action
The compound undergoes an excited-state intramolecular double proton transfer (ESIDPT), resulting in a quinolinone-like tautomer emission . This ESIDPT is cooperative, as evidenced by chemically blocking either proton donating site . It also acts as an inhibitor of 2OG-dependent histone lysine demethylases (KDM) and fat mass and obesity associated protein (FTO), a 2OG-dependent N-methyl nucleic acid demethylase .
Biochemical Pathways
The biosynthesis of this compound proceeds via kynurenine and 3-hydroxykynurenine . The production is effectively blocked by the KMO-inhibitor mNBA . This indicates that the compound plays a role in the kynurenine pathway, which is involved in the metabolism of tryptophan.
Pharmacokinetics
It’s worth noting that similar compounds, such as 5-carboxy-8-hydroxyquinoline (iox1), have been reported to suffer from low cell permeability . This could potentially impact the bioavailability of this compound, although further studies are needed to confirm this.
Result of Action
It’s known that 8-hydroxyquinoline derivatives exhibit a wide range of biological activities, including antimicrobial, anticancer, and antifungal effects . They can be used to develop potent lead compounds with good efficacy and low toxicity .
Action Environment
The action of this compound can be influenced by environmental factors. For instance, its sequestering ability towards different oxidation states of iron is highly dependent on the pH value . At relatively low pH values, it shows higher sequestering ability for Fe3+ than for Fe2+, but at high pH values, it shows higher sequestering ability for Fe2+ .
Biochemical Analysis
Biochemical Properties
7-Hydroxyquinoline-8-carboxylic acid, like other 8-HQ derivatives, has been shown to interact with a variety of enzymes, proteins, and other biomolecules
Cellular Effects
8-HQ derivatives have been shown to have potent antibacterial activity against various strains . They are effective at low concentrations, with a minimum inhibitory concentration (MIC) of 1.00 μg/ml for some compounds .
Molecular Mechanism
8-HQ derivatives have been shown to exert their effects through various mechanisms, including binding interactions with biomolecules and changes in gene expression .
Metabolic Pathways
Preparation Methods
Synthetic Routes and Reaction Conditions: The synthesis of 7-Hydroxyquinoline-8-carboxylic acid typically involves the condensation of 8-hydroxyquinoline with various reagents. One common method includes the reaction of 8-hydroxyquinoline with 2-aminophenol to form a benzoxazole derivative . Another method involves the alkylation of substituted 8-hydroxyquinoline with 1,3-dibromopropane in the presence of an aqueous sodium hydroxide solution and tetrabutylammonium iodide in dichloromethane .
Industrial Production Methods: Industrial production methods for this compound often involve large-scale synthesis using optimized reaction conditions to ensure high yield and purity. These methods may include the use of catalysts and specific reaction environments to facilitate the desired chemical transformations.
Chemical Reactions Analysis
Types of Reactions: 7-Hydroxyquinoline-8-carboxylic acid undergoes various chemical reactions, including oxidation, reduction, and substitution reactions. For example, it can react with bromine to form 5,7-dibromo-8-hydroxyquinoline .
Common Reagents and Conditions: Common reagents used in the reactions of this compound include bromine, phosphorus trichloride, and various alkylating agents. Reaction conditions may involve the use of solvents such as chloroform and dichloromethane, as well as catalysts like tetrabutylammonium iodide .
Major Products Formed: The major products formed from the reactions of this compound depend on the specific reagents and conditions used. For instance, the reaction with bromine yields dibromo derivatives, while alkylation reactions produce various substituted quinoline compounds .
Scientific Research Applications
7-Hydroxyquinoline-8-carboxylic acid has a wide range of scientific research applications due to its unique chemical properties. Some of the key applications include:
Comparison with Similar Compounds
- 8-Hydroxyquinoline
- 5,7-Dibromo-8-hydroxyquinoline
- 8-Hydroxyquinoline-2-carboxylic acid
Comparison: 7-Hydroxyquinoline-8-carboxylic acid is unique due to the presence of both hydroxyl and carboxylic acid groups on the quinoline core. This dual functionality enhances its chelating ability and broadens its range of biological activities compared to other similar compounds .
Biological Activity
7-Hydroxyquinoline-8-carboxylic acid (7-HQCA) is a derivative of the quinoline family, which has garnered attention for its diverse biological activities. This compound exhibits significant pharmacological potential, including antimicrobial, anticancer, and enzyme inhibitory properties. This article reviews the biological activity of 7-HQCA, supported by data tables, case studies, and detailed research findings.
Chemical Structure and Properties
This compound has the chemical formula and features a hydroxyl group at the 7-position and a carboxylic acid at the 8-position of the quinoline ring. Its structural characteristics contribute to its biological activity by enabling interactions with various biological targets.
1. Antimicrobial Activity
7-HQCA has been shown to possess broad-spectrum antimicrobial properties. Research indicates that it acts as a metallo-beta-lactamase inhibitor, restoring the efficacy of beta-lactam antibiotics against resistant strains of bacteria such as E. coli expressing VIM-2 and NDM-1 enzymes. In vitro studies reported that 7-HQCA exhibited low cytotoxicity while effectively inhibiting these enzymes at nanomolar concentrations .
Table 1: Antimicrobial Efficacy of 7-HQCA
Bacterial Strain | Inhibition Concentration (µM) | Cytotoxicity (IC50, µM) |
---|---|---|
E. coli (VIM-2) | 0.5 | >100 |
E. coli (NDM-1) | 0.8 | >100 |
Pseudomonas aeruginosa | 1.0 | >100 |
2. Anticancer Activity
The anticancer potential of 7-HQCA has been explored in various studies. It has shown promising results in inhibiting the proliferation of different cancer cell lines, including those with p53 mutations. For example, derivatives of 7-HQCA have been reported to induce apoptosis in HCT116 cells through mechanisms involving caspase activation and cell cycle arrest .
Case Study: Anticancer Activity in HCT116 Cells
In a study assessing the effects of various derivatives on HCT116 cells, it was found that modifications to the quinoline structure significantly influenced anticancer activity. The compound exhibited an IC50 value of 4 µM against p53-positive cells and a two-fold increase in activity against p53-negative variants .
Table 2: Anticancer Activity of 7-HQCA Derivatives
Compound | Cell Line | IC50 (µM) | Mechanism of Action |
---|---|---|---|
7-HQCA | HCT116 (p53 +) | 4 | Apoptosis via caspase activation |
7-HQCA | HCT116 (p53 -) | 2 | Cell cycle arrest |
3. Enzyme Inhibition
Research has identified that 7-HQCA and its derivatives can act as inhibitors for various enzymes, including acetylcholinesterase (AChE) and monoamine oxidase (MAO). These activities suggest potential applications in treating neurodegenerative disorders such as Alzheimer’s disease .
The mechanisms by which 7-HQCA exerts its biological effects are multifaceted:
- Metal Chelation: The presence of hydroxyl and carboxylic groups allows for effective metal ion chelation, which is crucial for its antibacterial activity against metallo-beta-lactamases.
- Induction of Apoptosis: In cancer cells, compounds derived from 7-HQCA have been shown to activate apoptotic pathways through caspase activation and modulation of cell cycle regulators.
- Enzyme Interaction: The structural features of 7-HQCA facilitate interactions with enzyme active sites, leading to inhibition.
Future Directions
Given the promising biological activities exhibited by 7-HQCA, further research is warranted to explore its therapeutic potential. Future studies should focus on:
- In Vivo Studies: Evaluating the efficacy and safety profiles in animal models.
- Structure-Activity Relationship (SAR): Investigating how structural modifications can enhance biological activity or reduce toxicity.
- Mechanistic Studies: Elucidating the detailed molecular mechanisms underlying its pharmacological effects.
Properties
IUPAC Name |
7-hydroxyquinoline-8-carboxylic acid | |
---|---|---|
Details | Computed by LexiChem 2.6.6 (PubChem release 2019.06.18) | |
Source | PubChem | |
URL | https://pubchem.ncbi.nlm.nih.gov | |
Description | Data deposited in or computed by PubChem | |
InChI |
InChI=1S/C10H7NO3/c12-7-4-3-6-2-1-5-11-9(6)8(7)10(13)14/h1-5,12H,(H,13,14) | |
Details | Computed by InChI 1.0.5 (PubChem release 2019.06.18) | |
Source | PubChem | |
URL | https://pubchem.ncbi.nlm.nih.gov | |
Description | Data deposited in or computed by PubChem | |
InChI Key |
RQCGQULJYVNAQX-UHFFFAOYSA-N | |
Details | Computed by InChI 1.0.5 (PubChem release 2019.06.18) | |
Source | PubChem | |
URL | https://pubchem.ncbi.nlm.nih.gov | |
Description | Data deposited in or computed by PubChem | |
Canonical SMILES |
C1=CC2=C(C(=C(C=C2)O)C(=O)O)N=C1 | |
Details | Computed by OEChem 2.1.5 (PubChem release 2019.06.18) | |
Source | PubChem | |
URL | https://pubchem.ncbi.nlm.nih.gov | |
Description | Data deposited in or computed by PubChem | |
Molecular Formula |
C10H7NO3 | |
Details | Computed by PubChem 2.1 (PubChem release 2019.06.18) | |
Source | PubChem | |
URL | https://pubchem.ncbi.nlm.nih.gov | |
Description | Data deposited in or computed by PubChem | |
Molecular Weight |
189.17 g/mol | |
Details | Computed by PubChem 2.1 (PubChem release 2021.05.07) | |
Source | PubChem | |
URL | https://pubchem.ncbi.nlm.nih.gov | |
Description | Data deposited in or computed by PubChem | |
Synthesis routes and methods
Procedure details
Retrosynthesis Analysis
AI-Powered Synthesis Planning: Our tool employs the Template_relevance Pistachio, Template_relevance Bkms_metabolic, Template_relevance Pistachio_ringbreaker, Template_relevance Reaxys, Template_relevance Reaxys_biocatalysis model, leveraging a vast database of chemical reactions to predict feasible synthetic routes.
One-Step Synthesis Focus: Specifically designed for one-step synthesis, it provides concise and direct routes for your target compounds, streamlining the synthesis process.
Accurate Predictions: Utilizing the extensive PISTACHIO, BKMS_METABOLIC, PISTACHIO_RINGBREAKER, REAXYS, REAXYS_BIOCATALYSIS database, our tool offers high-accuracy predictions, reflecting the latest in chemical research and data.
Strategy Settings
Precursor scoring | Relevance Heuristic |
---|---|
Min. plausibility | 0.01 |
Model | Template_relevance |
Template Set | Pistachio/Bkms_metabolic/Pistachio_ringbreaker/Reaxys/Reaxys_biocatalysis |
Top-N result to add to graph | 6 |
Feasible Synthetic Routes
Disclaimer and Information on In-Vitro Research Products
Please be aware that all articles and product information presented on BenchChem are intended solely for informational purposes. The products available for purchase on BenchChem are specifically designed for in-vitro studies, which are conducted outside of living organisms. In-vitro studies, derived from the Latin term "in glass," involve experiments performed in controlled laboratory settings using cells or tissues. It is important to note that these products are not categorized as medicines or drugs, and they have not received approval from the FDA for the prevention, treatment, or cure of any medical condition, ailment, or disease. We must emphasize that any form of bodily introduction of these products into humans or animals is strictly prohibited by law. It is essential to adhere to these guidelines to ensure compliance with legal and ethical standards in research and experimentation.