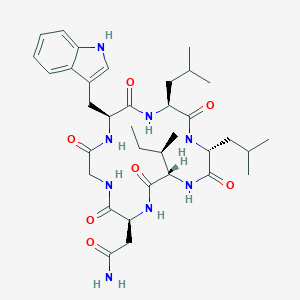
Desotamide
Overview
Description
Desotamide is a family of cyclohexapeptides primarily produced by the deep-sea-derived bacterium Streptomyces scopuliridis SCSIO ZJ46 . The founding member, this compound A, exhibits potent antibacterial activity against Gram-positive pathogens, including Streptococcus pneumoniae, Staphylococcus aureus, and methicillin-resistant Staphylococcus epidermidis (MRSE), with minimal inhibitory concentrations (MICs) ranging from 16 to 64 µg/mL . Structurally, desotamides are characterized by a cyclic backbone of six amino acids, with conserved residues such as tryptophan (Trp) at position V and glycine (Gly) at position VI . Modifications at specific amino acid positions (e.g., substitutions with D-amino acids or hydrophobic residues) significantly influence their bioactivity and selectivity . Notably, desotamides are noncytotoxic to mammalian cells (IC₅₀ > 30 µM), making them promising candidates for antibiotic development .
Preparation Methods
Synthetic Routes and Reaction Conditions: Desotamide is synthesized through non-ribosomal peptide synthetases (NRPSs), which are large multifunctional enzymes organized into modules . These modules incorporate single monomeric building blocks until the final polypeptide is generated . The biosynthesis involves loading, elongation, and termination modules, each contributing to the formation of the cyclic peptide structure .
Industrial Production Methods: Industrial production of this compound involves fermentation processes using Streptomyces species, which are known for producing a wide range of secondary metabolites . The fermentation conditions are optimized to enhance the yield of this compound, and the compound is subsequently purified using chromatographic techniques .
Chemical Reactions Analysis
Types of Reactions: Desotamide undergoes various chemical reactions, including oxidation, reduction, and substitution reactions . These reactions are essential for modifying the structure of this compound to enhance its antibacterial activity.
Common Reagents and Conditions: Common reagents used in the chemical reactions of this compound include oxidizing agents, reducing agents, and nucleophiles . The reactions are typically carried out under controlled conditions to ensure the desired modifications are achieved.
Major Products Formed: The major products formed from the chemical reactions of this compound include analogues with improved antibacterial activity . These analogues are synthesized by substituting specific amino acid residues in the cyclic peptide structure .
Scientific Research Applications
Antibacterial Activity
Desotamide exhibits notable antibacterial activity against a range of Gram-positive pathogens, including methicillin-resistant Staphylococcus aureus (MRSA) and other clinically relevant strains. Recent studies have highlighted the efficacy of this compound and its analogues in overcoming bacterial resistance:
- This compound A and its derivatives (e.g., desotamides A4 and A6) have shown a 2-4 fold increase in antibacterial activity compared to the original compound, with minimum inhibitory concentration (MIC) values ranging from 8 to 32 μg/mL against MRSA and other pathogens such as Streptococcus pneumoniae and Bacillus subtilis .
- The structure-activity relationship (SAR) studies indicate that modifications to the peptide structure significantly enhance its antibacterial properties. For instance, substitutions at specific amino acid positions have been linked to improved activity against resistant bacterial strains .
Potential for Drug Development
This compound's unique structure and mechanism of action make it a promising candidate for further pharmaceutical development:
- Non-cytotoxicity : Desotamides have been shown to be non-cytotoxic to mammalian cell lines at concentrations exceeding 30 μM, indicating a selective toxicity towards bacterial cells . This selectivity is crucial for developing antibiotics that minimize adverse effects on human cells.
- Broad-spectrum activity : The ability of desotamides to target multiple Gram-positive bacteria positions them as versatile agents in antibiotic therapy, particularly in an era where antibiotic resistance is a growing concern .
Case Studies and Research Findings
Several studies have documented the antibacterial efficacy of this compound and its derivatives:
Mechanism of Action
Desotamide exerts its antibacterial effects by targeting specific molecular pathways in bacterial cells . The compound interferes with the synthesis of bacterial cell walls, leading to cell lysis and death . The exact molecular targets and pathways involved in the mechanism of action of this compound are still under investigation, but it is known to disrupt essential processes in bacterial cells .
Comparison with Similar Compounds
Desotamides belong to a broader class of cyclic hexapeptides, including structurally related compounds such as wollamides, surugamides, and other synthetic analogues. Below is a detailed comparison based on structural features, bioactivity, and structure-activity relationships (SAR).
Structural Analogues: Wollamides
Wollamides (e.g., wollamides A, B, and their derivatives) are cyclic hexapeptides produced by soil-derived Streptomyces spp. . Key differences include:
- Position VI: Wollamides contain the basic amino acid ornithine (D-Orn) at position VI, whereas desotamides typically have glycine (Gly) .
- Bioactivity : Wollamides exhibit activity against mycobacteria (e.g., Mycobacterium tuberculosis), a feature absent in desotamides .
- Synthetic Modifications : Replacing D-Orn in wollamide B with D-Arg or D-Lys (yielding wollamide B1 and B2) enhances anti-Gram-positive activity by 2–4-fold (MIC: 8–32 µg/mL), mirroring the SAR observed in desotamides .
Natural Desotamide Analogues
Desotamides A–G vary in amino acid composition and bioactivity:
- Desotamides A and B : Active against MRSA and MRSE (MIC: 16–64 µg/mL) due to the presence of Trp at position V .
- Desotamides C and D : Inactive due to the absence of Trp at position V, confirming its indispensability for antibacterial activity .
- This compound G : Synthesized via heterologous expression in Streptomyces coelicolor M1152, this analogue lacks antibacterial activity (MIC > 113 µg/mL), highlighting the sensitivity of position I to substitutions .
Table 1: Antibacterial Activity of Selected this compound Analogues
Compound | Position II | Position VI | MIC (µg/mL) vs. MRSA | Key Feature |
---|---|---|---|---|
This compound A (1) | L-allo-Ile | Gly | 16–64 | Natural lead compound |
This compound A4 (13) | L-Ile | D-Lys | 8–32 | 2–4× improved activity |
This compound A6 (15) | L-Ile | D-Arg | 8–32 | Broad-spectrum Gram-positive |
Wollamide B (7) | L-Val | D-Orn | 16–64 | Anti-mycobacterial activity |
Wollamide B1 (8) | L-Val | D-Arg | 8–32 | Enhanced potency vs. B |
Toxicity and Selectivity
Desotamides and wollamides share low cytotoxicity (IC₅₀ > 30 µM against mammalian cell lines), indicating bacterial selectivity . This contrasts with glycopeptide antibiotics like vancomycin, which can cause nephrotoxicity .
Biological Activity
Desotamide is a cyclohexapeptide antibiotic produced by the marine-derived bacterium Streptomyces scopuliridis SCSIO ZJ46. This compound has garnered significant attention due to its potent antibacterial properties, particularly against drug-resistant strains of bacteria. This article explores the biological activity of this compound, focusing on its antibacterial efficacy, structure-activity relationships (SAR), and potential applications in combating antibiotic resistance.
Antibacterial Efficacy
This compound demonstrates notable antibacterial activity against various Gram-positive bacteria, including Streptococcus pneumoniae, Staphylococcus aureus, and methicillin-resistant Staphylococcus epidermidis (MRSE). The minimum inhibitory concentration (MIC) values for this compound against these pathogens range from 8 to 32 μg/mL, indicating its effectiveness, especially against MRSA, a prevalent clinical pathogen resistant to many antibiotics .
Table 1: Antibacterial Activity of this compound and Its Analogues
Compound | Target Bacteria | MIC (μg/mL) |
---|---|---|
This compound A | S. pneumoniae | 16 |
S. aureus | 8 | |
MRSE | 32 | |
This compound A4 | S. pneumoniae | 8 |
S. aureus | 8 | |
MRSA | 16 | |
This compound A6 | S. pneumoniae | 16 |
S. aureus | 8 | |
MRSA | 16 |
Structure-Activity Relationship (SAR)
The SAR studies of this compound have revealed that specific amino acid residues significantly influence its antibacterial activity. Notably, modifications at positions II and VI of the peptide chain enhance the antibacterial properties of this compound analogues. For instance, desotamides A4 and A6 exhibited a 2-4 fold increase in activity compared to the original this compound A when l-allo-Ile at position II was substituted with l-Ile and Gly at position VI was replaced by d-Lys or d-Arg .
Key Findings from SAR Studies:
- Position II : Substitution with l-Ile enhances activity.
- Position VI : Replacing Gly with d-Lys or d-Arg significantly boosts antibacterial efficacy.
- Trp Residue : The presence of tryptophan at position V is essential for maintaining bioactivity.
Although the precise mechanism by which this compound exerts its antibacterial effects remains to be fully elucidated, it is hypothesized that it disrupts bacterial cell wall synthesis or interferes with protein synthesis pathways. This disruption is critical in combating bacterial infections, particularly those caused by resistant strains .
Case Studies
Recent research has highlighted the potential of this compound and its analogues as promising candidates for new antibiotic therapies:
-
Study on this compound A4 and A6 :
- Conducted by researchers at the University of Queensland, this study synthesized multiple analogues of this compound and evaluated their antibacterial activities against a panel of pathogens.
- Results indicated that modifications led to significant enhancements in antibacterial potency, particularly against MRSA .
- Exploration of Biosynthetic Pathways :
Q & A
Basic Research Questions
Q. What experimental approaches are recommended for elucidating the biosynthesis pathway of Desotamide and its analogues?
To investigate this compound biosynthesis, combine genomic analysis of the Streptomyces biosynthetic gene cluster (BGC) with heterologous expression in model organisms like Streptomyces coelicolor. Key steps include:
- Gene cluster identification : Use bioinformatic tools (e.g., antiSMASH) to locate BGCs and compare homologous clusters (e.g., wolG1/wolG2 duplications in wollamide BGCs) .
- Heterologous expression : Express candidate BGCs in compatible hosts to validate peptide production and identify intermediates .
- Biochemical assays : Analyze enzyme specificity (e.g., adenylation domains in nonribosomal peptide synthetases) to confirm amino acid incorporation .
Q. How should researchers design this compound analogues to study structure-activity relationships (SAR)?
Prioritize systematic substitution at positions critical for antibacterial activity:
- Position V (Trp) : Essential for activity; substitutions here often abolish antimicrobial effects .
- Position VI : Replace Gly with basic residues (e.g., ornithine) to explore selectivity shifts (e.g., wollamide-like activity against mycobacteria) .
- Position I : Conservative substitutions (e.g., hydrophobic residues) to maintain backbone stability . Use solid-phase peptide synthesis (SPPS) or genetic engineering for analogue generation, followed by minimum inhibitory concentration (MIC) assays against Gram-positive pathogens .
Q. What are standard assays for evaluating this compound’s antibacterial activity and cytotoxicity?
- Antibacterial assays : Perform MIC tests against Staphylococcus aureus, Streptococcus pneumoniae, and methicillin-resistant strains (e.g., MRSE) using broth microdilution .
- Cytotoxicity screening : Test analogues against mammalian cell lines (e.g., HEK293) at concentrations >30 µM to confirm selectivity .
- Data interpretation : Compare activity trends across analogues (e.g., this compound A/B vs. C/D) to infer SAR .
Advanced Research Questions
Q. How can conflicting MIC data between this compound analogues be resolved?
Address contradictions by:
- Standardizing assays : Use consistent inoculum sizes, growth media, and endpoint criteria (e.g., optical density vs. colony counting) .
- Validating purity : Confirm analogue identity via LC-HRMS and NMR, as impurities (e.g., residual solvents) may skew results .
- Replicating studies : Compare results across independent labs to distinguish biological variability from methodological artifacts .
Q. What strategies optimize heterologous expression of this compound BGCs in non-native hosts?
- Promoter engineering : Use strong, constitutive promoters (e.g., ermE) to enhance transcription in S. coelicolor .
- Co-expression of chaperones : Improve folding of large NRPS proteins .
- Fermentation optimization : Adjust pH, temperature, and nutrient availability to maximize peptide yield .
Q. How should researchers integrate multi-omics data to refine this compound’s SAR?
Combine:
- Transcriptomics : Identify BGC expression patterns under stress conditions (e.g., nutrient limitation) .
- Metabolomics : Correlate analogue production with specific growth phases using LC-MS/MS .
- Structural modeling : Predict binding modes via molecular docking against bacterial membrane targets (e.g., lipid II) .
Q. What methodologies address the unknown antimicrobial target of this compound?
- Chemical proteomics : Use photoaffinity-labeled this compound analogues to capture interacting proteins .
- Genomic mutagenesis : Screen for resistant bacterial mutants and sequence mutations to identify target genes .
- Membrane disruption assays : Measure dye leakage from liposomes to test pore-forming activity .
Q. Methodological Best Practices
Q. What are key considerations for ensuring reproducible synthesis of this compound analogues?
- Documentation : Provide detailed protocols for SPPS (e.g., coupling reagents, cleavage conditions) in supplementary materials .
- Characterization : Report exact LC-HRMS/NMR parameters (e.g., solvent systems, column types) for analogue verification .
- Negative controls : Include non-active analogues (e.g., this compound G) to validate assay specificity .
Q. How can researchers reconcile discrepancies in BGC annotation for this compound-producing strains?
- Comparative genomics : Align BGCs from multiple Streptomyces strains to identify conserved domains .
- Gene knockout : Disrupt candidate genes (e.g., dsaG) to confirm their role in biosynthesis .
- Phylogenetic analysis : Cluster BGCs with known pathways to infer evolutionary relationships .
Q. Data Presentation & Literature Evaluation
Q. What frameworks support rigorous interpretation of this compound’s bioactivity data?
- Dose-response modeling : Calculate EC₅₀ values for cytotoxicity vs. antimicrobial activity to quantify selectivity .
- Meta-analysis : Aggregate published MIC data to identify consensus trends (e.g., Trp indispensability) .
- Confounder adjustment : Control for variables like bacterial growth phase in assay design .
Properties
IUPAC Name |
2-[(2S,8S,11S,14R,17S)-17-[(2R)-butan-2-yl]-8-(1H-indol-3-ylmethyl)-11,14-bis(2-methylpropyl)-3,6,9,12,15,18-hexaoxo-1,4,7,10,13,16-hexazacyclooctadec-2-yl]acetamide | |
---|---|---|
Source | PubChem | |
URL | https://pubchem.ncbi.nlm.nih.gov | |
Description | Data deposited in or computed by PubChem | |
InChI |
InChI=1S/C35H52N8O7/c1-7-20(6)30-35(50)42-27(15-28(36)44)31(46)38-17-29(45)39-26(14-21-16-37-23-11-9-8-10-22(21)23)33(48)40-24(12-18(2)3)32(47)41-25(13-19(4)5)34(49)43-30/h8-11,16,18-20,24-27,30,37H,7,12-15,17H2,1-6H3,(H2,36,44)(H,38,46)(H,39,45)(H,40,48)(H,41,47)(H,42,50)(H,43,49)/t20-,24+,25-,26+,27+,30+/m1/s1 | |
Source | PubChem | |
URL | https://pubchem.ncbi.nlm.nih.gov | |
Description | Data deposited in or computed by PubChem | |
InChI Key |
LJGXNPVJAKBNOK-DTWKRICWSA-N | |
Source | PubChem | |
URL | https://pubchem.ncbi.nlm.nih.gov | |
Description | Data deposited in or computed by PubChem | |
Canonical SMILES |
CCC(C)C1C(=O)NC(C(=O)NCC(=O)NC(C(=O)NC(C(=O)NC(C(=O)N1)CC(C)C)CC(C)C)CC2=CNC3=CC=CC=C32)CC(=O)N | |
Source | PubChem | |
URL | https://pubchem.ncbi.nlm.nih.gov | |
Description | Data deposited in or computed by PubChem | |
Isomeric SMILES |
CC[C@@H](C)[C@H]1C(=O)N[C@H](C(=O)NCC(=O)N[C@H](C(=O)N[C@H](C(=O)N[C@@H](C(=O)N1)CC(C)C)CC(C)C)CC2=CNC3=CC=CC=C32)CC(=O)N | |
Source | PubChem | |
URL | https://pubchem.ncbi.nlm.nih.gov | |
Description | Data deposited in or computed by PubChem | |
Molecular Formula |
C35H52N8O7 | |
Source | PubChem | |
URL | https://pubchem.ncbi.nlm.nih.gov | |
Description | Data deposited in or computed by PubChem | |
DSSTOX Substance ID |
DTXSID70941214 | |
Record name | 2-[17-(Butan-2-yl)-3,6,9,12,15,18-hexahydroxy-8-[(1H-indol-3-yl)methyl]-11,14-bis(2-methylpropyl)-1,4,7,10,13,16-hexaazacyclooctadeca-3,6,9,12,15,18-hexaen-2-yl]ethanimidic acid | |
Source | EPA DSSTox | |
URL | https://comptox.epa.gov/dashboard/DTXSID70941214 | |
Description | DSSTox provides a high quality public chemistry resource for supporting improved predictive toxicology. | |
Molecular Weight |
696.8 g/mol | |
Source | PubChem | |
URL | https://pubchem.ncbi.nlm.nih.gov | |
Description | Data deposited in or computed by PubChem | |
CAS No. |
194660-14-5 | |
Record name | Desotamide | |
Source | ChemIDplus | |
URL | https://pubchem.ncbi.nlm.nih.gov/substance/?source=chemidplus&sourceid=0194660145 | |
Description | ChemIDplus is a free, web search system that provides access to the structure and nomenclature authority files used for the identification of chemical substances cited in National Library of Medicine (NLM) databases, including the TOXNET system. | |
Record name | 2-[17-(Butan-2-yl)-3,6,9,12,15,18-hexahydroxy-8-[(1H-indol-3-yl)methyl]-11,14-bis(2-methylpropyl)-1,4,7,10,13,16-hexaazacyclooctadeca-3,6,9,12,15,18-hexaen-2-yl]ethanimidic acid | |
Source | EPA DSSTox | |
URL | https://comptox.epa.gov/dashboard/DTXSID70941214 | |
Description | DSSTox provides a high quality public chemistry resource for supporting improved predictive toxicology. | |
Retrosynthesis Analysis
AI-Powered Synthesis Planning: Our tool employs the Template_relevance Pistachio, Template_relevance Bkms_metabolic, Template_relevance Pistachio_ringbreaker, Template_relevance Reaxys, Template_relevance Reaxys_biocatalysis model, leveraging a vast database of chemical reactions to predict feasible synthetic routes.
One-Step Synthesis Focus: Specifically designed for one-step synthesis, it provides concise and direct routes for your target compounds, streamlining the synthesis process.
Accurate Predictions: Utilizing the extensive PISTACHIO, BKMS_METABOLIC, PISTACHIO_RINGBREAKER, REAXYS, REAXYS_BIOCATALYSIS database, our tool offers high-accuracy predictions, reflecting the latest in chemical research and data.
Strategy Settings
Precursor scoring | Relevance Heuristic |
---|---|
Min. plausibility | 0.01 |
Model | Template_relevance |
Template Set | Pistachio/Bkms_metabolic/Pistachio_ringbreaker/Reaxys/Reaxys_biocatalysis |
Top-N result to add to graph | 6 |
Feasible Synthetic Routes
Disclaimer and Information on In-Vitro Research Products
Please be aware that all articles and product information presented on BenchChem are intended solely for informational purposes. The products available for purchase on BenchChem are specifically designed for in-vitro studies, which are conducted outside of living organisms. In-vitro studies, derived from the Latin term "in glass," involve experiments performed in controlled laboratory settings using cells or tissues. It is important to note that these products are not categorized as medicines or drugs, and they have not received approval from the FDA for the prevention, treatment, or cure of any medical condition, ailment, or disease. We must emphasize that any form of bodily introduction of these products into humans or animals is strictly prohibited by law. It is essential to adhere to these guidelines to ensure compliance with legal and ethical standards in research and experimentation.