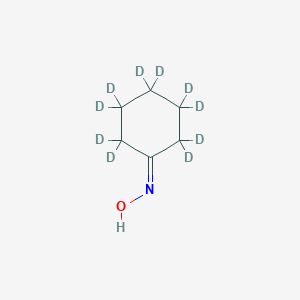
Cyclohexanone-D10-oxime
Overview
Description
Cyclohexanone-D10-oxime is a deuterated derivative of cyclohexanone oxime, where all hydrogen atoms are replaced by deuterium. This compound is primarily used in scientific research due to its unique isotopic properties, which make it valuable for various analytical and synthetic applications.
Preparation Methods
Synthetic Routes and Reaction Conditions: Cyclohexanone-D10-oxime can be synthesized through the nucleophilic addition of deuterated hydroxylamine to cyclohexanone-D10. The reaction typically involves the following steps:
Formation of Deuterated Hydroxylamine: Deuterated hydroxylamine can be prepared by the reduction of deuterated nitro compounds using deuterium gas.
Reaction with Cyclohexanone-D10: The deuterated hydroxylamine is then reacted with cyclohexanone-D10 under mild acidic conditions to form this compound.
Industrial Production Methods: The industrial production of this compound follows similar synthetic routes but on a larger scale. The process involves the use of deuterated reagents and catalysts to ensure high yield and purity of the final product.
Chemical Reactions Analysis
Types of Reactions: Cyclohexanone-D10-oxime undergoes various chemical reactions, including:
Oxidation: It can be oxidized to form deuterated nitroso compounds.
Reduction: It can be reduced to form deuterated amines.
Substitution: It can undergo substitution reactions with various nucleophiles.
Common Reagents and Conditions:
Oxidation: Common oxidizing agents include potassium permanganate and hydrogen peroxide.
Reduction: Common reducing agents include lithium aluminum hydride and sodium borohydride.
Substitution: Common nucleophiles include halides and alkoxides.
Major Products:
Oxidation: Deuterated nitroso compounds.
Reduction: Deuterated amines.
Substitution: Various substituted deuterated cyclohexanone derivatives.
Scientific Research Applications
Cyclohexanone-D10-oxime has a wide range of applications in scientific research:
Chemistry: It is used as a precursor in the synthesis of deuterated compounds and as a reagent in isotopic labeling studies.
Biology: It is used in metabolic studies to trace the pathways of deuterated compounds in biological systems.
Medicine: It is used in the development of deuterated drugs, which often exhibit improved pharmacokinetic properties.
Industry: It is used in the production of deuterated polymers and other materials with unique properties.
Mechanism of Action
The mechanism of action of cyclohexanone-D10-oxime involves its interaction with various molecular targets and pathways:
Formation of Deuterated Intermediates: The compound can form deuterated intermediates through nucleophilic addition and substitution reactions.
Pathways Involved: The deuterated intermediates can participate in various biochemical pathways, leading to the formation of deuterated metabolites.
Comparison with Similar Compounds
Cyclohexanone-D10-oxime can be compared with other similar compounds, such as:
Cyclohexanone oxime: The non-deuterated version of the compound, which is commonly used in the production of Nylon-6.
Cyclohexanone-D10: The deuterated version of cyclohexanone, which is used in similar applications but lacks the oxime functional group.
Deuterated hydroxylamine: A precursor in the synthesis of this compound, which is used in various isotopic labeling studies.
Uniqueness: this compound is unique due to its deuterated nature, which makes it valuable for isotopic labeling and tracing studies. Its stability and reactivity also make it a versatile compound in synthetic and analytical chemistry.
Biological Activity
Cyclohexanone-D10-oxime, a deuterated derivative of cyclohexanone oxime, has garnered attention in scientific research due to its unique properties and potential biological activities. This article delves into the biological activity of this compound, examining its mutagenicity, toxicity, and pharmacokinetics based on various research findings.
This compound is chemically represented as C₆H₁₀D₁₀NO, with a molecular weight of approximately 123.22 g/mol. The compound is characterized by the presence of a hydroxylamine functional group attached to a cyclohexanone ring, which influences its reactivity and biological interactions.
Property | Value |
---|---|
Molecular Formula | C₆H₁₀D₁₀NO |
Molecular Weight | 123.22 g/mol |
CAS Number | 169297-52-3 |
Density | N/A |
Boiling Point | N/A |
Mutagenicity and Toxicity
Research indicates that cyclohexanone oxime exhibits mutagenic properties under certain conditions. A study evaluated its mutagenicity using the Salmonella typhimurium assay, where it showed positive results in strain TA1535 with S9 activation but negative results in other strains (TA97, TA98, TA100) both with and without S9 . The compound's potential to induce chromosomal aberrations was also assessed in cultured Chinese hamster ovary cells, yielding equivocal results without S9 activation and negative results with S9 .
Case Study: Toxicological Assessment
A comprehensive toxicological assessment was conducted over 13 weeks on mice administered varying concentrations of cyclohexanone oxime. Key findings included:
- No deaths were recorded at lower concentrations (up to 2,500 ppm).
- Significant increases in relative spleen weights were observed at exposure levels of 5,000 and 10,000 ppm.
- Hepatotoxic effects were noted at concentrations as low as 1,250 ppm for males and 2,500 ppm for females .
Pharmacokinetics
The pharmacokinetic profile of cyclohexanone oxime indicates rapid clearance from plasma following intravenous administration. The half-lives observed were approximately 1.6 minutes (alpha phase) and 18.2 minutes (beta phase) . When administered dermally, only about 4% to 5% of the dose was recovered in urine and tissues, with most volatilizing from the skin surface .
Table 2: Summary of Toxicological Findings
Exposure Level (ppm) | Observations |
---|---|
0 | Control group |
106 | No significant effects |
312 | Increased liver weight in males |
625 | Mild olfactory epithelial degeneration |
1,250 | Hepatotoxicity observed |
2,500 | Increased spleen weight; no deaths |
5,000 | Significant increases in spleen and liver weight; hematopoietic cell proliferation observed |
10,000 | Deaths occurred; severe liver and spleen effects |
Mechanistic Insights
Recent studies utilizing vibrational spectroscopy have provided insights into the molecular interactions and conformational changes of cyclohexanone oxime. These studies are crucial for understanding how the compound behaves under various conditions and can help elucidate its biological mechanisms .
In Situ Characterization
In situ characterization techniques such as infrared (IR) and Raman spectroscopy have been employed to analyze the vibrational spectra of cyclohexanone oxime. These studies reveal significant information about hydrogen bonding interactions and conformational stability across different phases .
Case Study: Vibrational Spectroscopy Analysis
A detailed investigation highlighted the vibrational modes associated with cyclohexanone oxime in solid-state forms. The research demonstrated that phase transitions could be tracked through specific spectral changes, providing a deeper understanding of the compound's structural dynamics during biological interactions .
Properties
IUPAC Name |
N-(2,2,3,3,4,4,5,5,6,6-decadeuteriocyclohexylidene)hydroxylamine | |
---|---|---|
Source | PubChem | |
URL | https://pubchem.ncbi.nlm.nih.gov | |
Description | Data deposited in or computed by PubChem | |
InChI |
InChI=1S/C6H11NO/c8-7-6-4-2-1-3-5-6/h8H,1-5H2/i1D2,2D2,3D2,4D2,5D2 | |
Source | PubChem | |
URL | https://pubchem.ncbi.nlm.nih.gov | |
Description | Data deposited in or computed by PubChem | |
InChI Key |
VEZUQRBDRNJBJY-YXALHFAPSA-N | |
Source | PubChem | |
URL | https://pubchem.ncbi.nlm.nih.gov | |
Description | Data deposited in or computed by PubChem | |
Canonical SMILES |
C1CCC(=NO)CC1 | |
Source | PubChem | |
URL | https://pubchem.ncbi.nlm.nih.gov | |
Description | Data deposited in or computed by PubChem | |
Isomeric SMILES |
[2H]C1(C(=NO)C(C(C(C1([2H])[2H])([2H])[2H])([2H])[2H])([2H])[2H])[2H] | |
Source | PubChem | |
URL | https://pubchem.ncbi.nlm.nih.gov | |
Description | Data deposited in or computed by PubChem | |
Molecular Formula |
C6H11NO | |
Source | PubChem | |
URL | https://pubchem.ncbi.nlm.nih.gov | |
Description | Data deposited in or computed by PubChem | |
Molecular Weight |
123.22 g/mol | |
Source | PubChem | |
URL | https://pubchem.ncbi.nlm.nih.gov | |
Description | Data deposited in or computed by PubChem | |
Retrosynthesis Analysis
AI-Powered Synthesis Planning: Our tool employs the Template_relevance Pistachio, Template_relevance Bkms_metabolic, Template_relevance Pistachio_ringbreaker, Template_relevance Reaxys, Template_relevance Reaxys_biocatalysis model, leveraging a vast database of chemical reactions to predict feasible synthetic routes.
One-Step Synthesis Focus: Specifically designed for one-step synthesis, it provides concise and direct routes for your target compounds, streamlining the synthesis process.
Accurate Predictions: Utilizing the extensive PISTACHIO, BKMS_METABOLIC, PISTACHIO_RINGBREAKER, REAXYS, REAXYS_BIOCATALYSIS database, our tool offers high-accuracy predictions, reflecting the latest in chemical research and data.
Strategy Settings
Precursor scoring | Relevance Heuristic |
---|---|
Min. plausibility | 0.01 |
Model | Template_relevance |
Template Set | Pistachio/Bkms_metabolic/Pistachio_ringbreaker/Reaxys/Reaxys_biocatalysis |
Top-N result to add to graph | 6 |
Feasible Synthetic Routes
Disclaimer and Information on In-Vitro Research Products
Please be aware that all articles and product information presented on BenchChem are intended solely for informational purposes. The products available for purchase on BenchChem are specifically designed for in-vitro studies, which are conducted outside of living organisms. In-vitro studies, derived from the Latin term "in glass," involve experiments performed in controlled laboratory settings using cells or tissues. It is important to note that these products are not categorized as medicines or drugs, and they have not received approval from the FDA for the prevention, treatment, or cure of any medical condition, ailment, or disease. We must emphasize that any form of bodily introduction of these products into humans or animals is strictly prohibited by law. It is essential to adhere to these guidelines to ensure compliance with legal and ethical standards in research and experimentation.