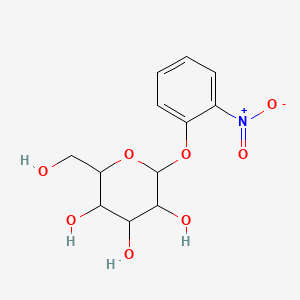
2-(hydroxymethyl)-6-(2-nitrophenoxy)oxane-3,4,5-triol
Overview
Description
2-(hydroxymethyl)-6-(2-nitrophenoxy)oxane-3,4,5-triol is a complex organic compound characterized by its unique structural features
Preparation Methods
Synthetic Routes and Reaction Conditions
The synthesis of 2-(hydroxymethyl)-6-(2-nitrophenoxy)oxane-3,4,5-triol typically involves multiple steps. One common method includes the reaction of a suitable oxane derivative with a nitrophenol compound under controlled conditions. The reaction is often catalyzed by acids or bases, depending on the specific requirements of the synthesis.
Industrial Production Methods
In an industrial setting, the production of this compound may involve large-scale batch reactors where the reactants are combined and subjected to specific temperature and pressure conditions to optimize yield and purity. The process may also include purification steps such as crystallization or chromatography to isolate the desired product.
Chemical Reactions Analysis
Types of Reactions
2-(hydroxymethyl)-6-(2-nitrophenoxy)oxane-3,4,5-triol can undergo various chemical reactions, including:
Oxidation: The hydroxymethyl group can be oxidized to form corresponding aldehydes or carboxylic acids.
Reduction: The nitro group can be reduced to an amino group under suitable conditions.
Substitution: The phenoxy group can participate in nucleophilic substitution reactions.
Common Reagents and Conditions
Oxidation: Common oxidizing agents include potassium permanganate (KMnO4) and chromium trioxide (CrO3).
Reduction: Reducing agents such as hydrogen gas (H2) in the presence of a palladium catalyst (Pd/C) are often used.
Substitution: Nucleophiles such as sodium methoxide (NaOCH3) can be employed for substitution reactions.
Major Products Formed
Oxidation: Formation of aldehydes or carboxylic acids.
Reduction: Formation of amino derivatives.
Substitution: Formation of substituted phenoxy derivatives.
Scientific Research Applications
2-(hydroxymethyl)-6-(2-nitrophenoxy)oxane-3,4,5-triol has a wide range of applications in scientific research:
Chemistry: Used as a building block for the synthesis of more complex molecules.
Biology: Investigated for its potential as a biochemical probe.
Medicine: Explored for its therapeutic potential in drug development.
Industry: Utilized in the production of specialty chemicals and materials.
Mechanism of Action
The mechanism of action of 2-(hydroxymethyl)-6-(2-nitrophenoxy)oxane-3,4,5-triol involves its interaction with specific molecular targets. The compound can bind to enzymes or receptors, modulating their activity and triggering downstream signaling pathways. The exact molecular targets and pathways depend on the specific application and context of use.
Comparison with Similar Compounds
Similar Compounds
- 2-(hydroxymethyl)-6-(2-aminophenoxy)oxane-3,4,5-triol
- 2-(hydroxymethyl)-6-(2-chlorophenoxy)oxane-3,4,5-triol
Uniqueness
2-(hydroxymethyl)-6-(2-nitrophenoxy)oxane-3,4,5-triol is unique due to its specific combination of functional groups, which confer distinct chemical reactivity and biological activity. This uniqueness makes it a valuable compound for various research and industrial applications.
Properties
IUPAC Name |
2-(hydroxymethyl)-6-(2-nitrophenoxy)oxane-3,4,5-triol | |
---|---|---|
Details | Computed by Lexichem TK 2.7.0 (PubChem release 2021.05.07) | |
Source | PubChem | |
URL | https://pubchem.ncbi.nlm.nih.gov | |
Description | Data deposited in or computed by PubChem | |
InChI |
InChI=1S/C12H15NO8/c14-5-8-9(15)10(16)11(17)12(21-8)20-7-4-2-1-3-6(7)13(18)19/h1-4,8-12,14-17H,5H2 | |
Details | Computed by InChI 1.0.6 (PubChem release 2021.05.07) | |
Source | PubChem | |
URL | https://pubchem.ncbi.nlm.nih.gov | |
Description | Data deposited in or computed by PubChem | |
InChI Key |
KUWPCJHYPSUOFW-UHFFFAOYSA-N | |
Details | Computed by InChI 1.0.6 (PubChem release 2021.05.07) | |
Source | PubChem | |
URL | https://pubchem.ncbi.nlm.nih.gov | |
Description | Data deposited in or computed by PubChem | |
Canonical SMILES |
C1=CC=C(C(=C1)[N+](=O)[O-])OC2C(C(C(C(O2)CO)O)O)O | |
Details | Computed by OEChem 2.3.0 (PubChem release 2021.05.07) | |
Source | PubChem | |
URL | https://pubchem.ncbi.nlm.nih.gov | |
Description | Data deposited in or computed by PubChem | |
Molecular Formula |
C12H15NO8 | |
Details | Computed by PubChem 2.1 (PubChem release 2021.05.07) | |
Source | PubChem | |
URL | https://pubchem.ncbi.nlm.nih.gov | |
Description | Data deposited in or computed by PubChem | |
Molecular Weight |
301.25 g/mol | |
Details | Computed by PubChem 2.1 (PubChem release 2021.05.07) | |
Source | PubChem | |
URL | https://pubchem.ncbi.nlm.nih.gov | |
Description | Data deposited in or computed by PubChem | |
CAS No. |
369-07-3, 2816-24-2 | |
Record name | Galactopyranoside, .beta.-D- | |
Source | DTP/NCI | |
URL | https://dtp.cancer.gov/dtpstandard/servlet/dwindex?searchtype=NSC&outputformat=html&searchlist=83631 | |
Description | The NCI Development Therapeutics Program (DTP) provides services and resources to the academic and private-sector research communities worldwide to facilitate the discovery and development of new cancer therapeutic agents. | |
Explanation | Unless otherwise indicated, all text within NCI products is free of copyright and may be reused without our permission. Credit the National Cancer Institute as the source. | |
Record name | Glucopyranoside, .beta.-D- | |
Source | DTP/NCI | |
URL | https://dtp.cancer.gov/dtpstandard/servlet/dwindex?searchtype=NSC&outputformat=html&searchlist=1947 | |
Description | The NCI Development Therapeutics Program (DTP) provides services and resources to the academic and private-sector research communities worldwide to facilitate the discovery and development of new cancer therapeutic agents. | |
Explanation | Unless otherwise indicated, all text within NCI products is free of copyright and may be reused without our permission. Credit the National Cancer Institute as the source. | |
Synthesis routes and methods
Procedure details
Retrosynthesis Analysis
AI-Powered Synthesis Planning: Our tool employs the Template_relevance Pistachio, Template_relevance Bkms_metabolic, Template_relevance Pistachio_ringbreaker, Template_relevance Reaxys, Template_relevance Reaxys_biocatalysis model, leveraging a vast database of chemical reactions to predict feasible synthetic routes.
One-Step Synthesis Focus: Specifically designed for one-step synthesis, it provides concise and direct routes for your target compounds, streamlining the synthesis process.
Accurate Predictions: Utilizing the extensive PISTACHIO, BKMS_METABOLIC, PISTACHIO_RINGBREAKER, REAXYS, REAXYS_BIOCATALYSIS database, our tool offers high-accuracy predictions, reflecting the latest in chemical research and data.
Strategy Settings
Precursor scoring | Relevance Heuristic |
---|---|
Min. plausibility | 0.01 |
Model | Template_relevance |
Template Set | Pistachio/Bkms_metabolic/Pistachio_ringbreaker/Reaxys/Reaxys_biocatalysis |
Top-N result to add to graph | 6 |
Feasible Synthetic Routes
Disclaimer and Information on In-Vitro Research Products
Please be aware that all articles and product information presented on BenchChem are intended solely for informational purposes. The products available for purchase on BenchChem are specifically designed for in-vitro studies, which are conducted outside of living organisms. In-vitro studies, derived from the Latin term "in glass," involve experiments performed in controlled laboratory settings using cells or tissues. It is important to note that these products are not categorized as medicines or drugs, and they have not received approval from the FDA for the prevention, treatment, or cure of any medical condition, ailment, or disease. We must emphasize that any form of bodily introduction of these products into humans or animals is strictly prohibited by law. It is essential to adhere to these guidelines to ensure compliance with legal and ethical standards in research and experimentation.