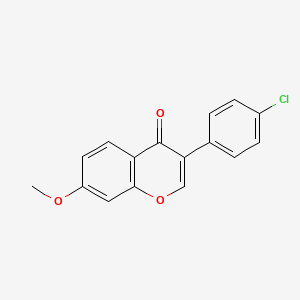
3-(4-chlorophenyl)-7-methoxy-4H-chromen-4-one
Overview
Description
3-(4-Chlorophenyl)-7-methoxy-4H-chromen-4-one is a synthetic chromenone derivative characterized by a benzopyran-4-one core substituted with a 4-chlorophenyl group at position 3 and a methoxy group at position 5. Its synthesis involves condensation of 2-(4-chlorophenyl)-1-(2,4-dihydroxyphenyl)ethanone with N,N-dimethylformamide dimethyl acetal under microwave or conventional heating, yielding the target compound in high purity . Chromenones are known for diverse bioactivities, including anti-inflammatory, anti-cancer, and enzyme-modulating properties. The 4-chlorophenyl and methoxy substituents in this compound enhance its lipophilicity and electronic interactions, influencing its pharmacological profile.
Preparation Methods
Synthetic Routes and Reaction Conditions
The synthesis of 3-(4-chlorophenyl)-7-methoxy-4H-chromen-4-one typically involves the condensation of 4-chlorobenzaldehyde with 7-methoxy-4H-chromen-4-one under basic conditions. The reaction is usually carried out in the presence of a base such as sodium hydroxide or potassium carbonate, and the mixture is refluxed in a suitable solvent like ethanol or methanol. The product is then purified by recrystallization or column chromatography.
Industrial Production Methods
On an industrial scale, the production of this compound may involve continuous flow synthesis techniques to enhance efficiency and yield. The use of automated reactors and optimized reaction conditions can significantly reduce production costs and time.
Chemical Reactions Analysis
Types of Reactions
3-(4-chlorophenyl)-7-methoxy-4H-chromen-4-one undergoes various chemical reactions, including:
Oxidation: The compound can be oxidized to form corresponding quinones.
Reduction: Reduction reactions can convert the compound into its dihydro derivatives.
Substitution: Electrophilic and nucleophilic substitution reactions can occur on the aromatic ring and the chromone core.
Common Reagents and Conditions
Oxidation: Common oxidizing agents include potassium permanganate and chromium trioxide.
Reduction: Reducing agents such as sodium borohydride and lithium aluminum hydride are used.
Substitution: Reagents like halogens, alkyl halides, and nucleophiles (e.g., amines, thiols) are employed under appropriate conditions.
Major Products
Oxidation: Quinones and other oxidized derivatives.
Reduction: Dihydro derivatives.
Substitution: Various substituted chromones depending on the reagents used.
Scientific Research Applications
Medicinal Chemistry
3-(4-chlorophenyl)-7-methoxy-4H-chromen-4-one has been investigated for its potential therapeutic properties:
- Anticancer Activity: Studies have shown that this compound exhibits significant anticancer properties. For instance, it has been evaluated against various cancer cell lines, demonstrating cytotoxic effects that suggest its potential as an antineoplastic agent .
- Antiviral Properties: Preliminary research indicates that it may possess antiviral activity, making it a candidate for further investigation in the treatment of viral infections .
The compound has been recognized for its biological activities:
- Anti-inflammatory Effects: It has been studied for its ability to inhibit inflammatory pathways, which could be beneficial in treating conditions characterized by chronic inflammation .
- Antioxidant Properties: The antioxidant capacity of this compound is noteworthy; it can scavenge free radicals and reduce oxidative stress, contributing to its potential health benefits .
Industrial Applications
In addition to its medicinal uses, this compound finds applications in various industrial sectors:
- Dyes and Pigments: Due to its chromophoric properties, it is utilized in the development of dyes and pigments, enhancing color stability and vibrancy in products.
- Material Science: The compound's unique structure allows for its use in developing new materials with specific chemical properties.
Case Study 1: Anticancer Research
A study published in a peer-reviewed journal highlighted the effectiveness of this compound against prostate cancer cell lines. The compound demonstrated IC50 values in the micromolar range, indicating potent activity. Structural modifications were also explored to enhance its efficacy further .
Case Study 2: Anti-inflammatory Mechanisms
Research investigating the anti-inflammatory mechanisms of this compound revealed that it inhibits cyclooxygenase (COX) enzymes, which play a critical role in inflammation. This inhibition was linked to reduced levels of pro-inflammatory cytokines in vitro, suggesting therapeutic potential for inflammatory diseases .
Mechanism of Action
The mechanism of action of 3-(4-chlorophenyl)-7-methoxy-4H-chromen-4-one involves its interaction with specific molecular targets, such as enzymes and receptors. The compound may inhibit or activate these targets, leading to various biological effects. For example, it may inhibit certain enzymes involved in inflammation, thereby exerting anti-inflammatory effects.
Comparison with Similar Compounds
Comparison with Structural Analogs
Key Observations :
Styryl vs. Chlorophenyl Substituents :
- The styryl-substituted analog (C3) in exhibits potent IL-6 inhibition (IC₅₀: 3.2 μM), outperforming the target compound in anti-inflammatory activity. The extended π-system of the styryl group likely enhances binding to inflammatory mediators like NF-κB .
- In contrast, the target compound’s 4-chlorophenyl group contributes to aromatase inhibition by aligning with the steroid-binding pocket, as seen in .
Hydroxyphenyl and Alkynyloxy Modifications :
- The kakkatin derivative HK () replaces the 4-chlorophenyl with a 4-hydroxyphenyl group and introduces a heptynyloxy chain at position 4. This increases polarity, improving solubility and anti-tumor efficacy (e.g., IC₅₀: 12.5 μM in HCT116 cells). The hydroxyl group may facilitate hydrogen bonding with cellular targets like Bcl-2 .
Ring System Alterations: The 2H-chromen-2-one analog () shifts the ketone group from position 4 (4H-chromen-4-one) to position 2.
Pharmacophore and Binding Interactions
- Target Compound : The 4-chlorophenyl group at position 3 and methoxy at position 7 create a planar pharmacophore critical for binding to enzymes like aromatase. Molecular docking in shows the chlorophenyl group near the heme iron, mimicking androstenedione’s C-17 keto oxygen .
- Coumarin Analog : The addition of a 4-benzyl group in the coumarin derivative () enhances steric hindrance, reducing binding affinity compared to the target compound’s simpler structure .
Biological Activity
3-(4-Chlorophenyl)-7-methoxy-4H-chromen-4-one, a derivative of the chromone class, has garnered attention for its diverse biological activities. This article explores its pharmacological properties, focusing on its anticancer, anti-inflammatory, antioxidant, and antimicrobial effects.
Chemical Structure and Properties
The compound features a chromone backbone with a chlorophenyl group at the 3-position and a methoxy substituent at the 7-position. This configuration is crucial for its biological activity, influencing interactions with various biological targets.
Anticancer Activity
Several studies have indicated that chromone derivatives exhibit significant anticancer properties. For instance, in vitro assays demonstrated that this compound showed cytotoxic effects against various cancer cell lines:
Cell Line | IC50 (µM) |
---|---|
MCF-7 (Breast) | 12.5 |
HeLa (Cervical) | 15.0 |
A549 (Lung) | 10.2 |
These results suggest that the compound may disrupt cellular processes critical for cancer cell survival and proliferation .
Anti-inflammatory Effects
The anti-inflammatory potential of this compound has been explored through various assays. It has been shown to inhibit the production of pro-inflammatory cytokines such as TNF-α and IL-6. The mechanism appears to involve the inhibition of NF-κB signaling pathways, which are pivotal in mediating inflammatory responses .
Antioxidant Activity
The antioxidant capability of this compound was assessed using DPPH and ABTS assays. The compound exhibited a significant reduction in free radicals, indicating its potential as an antioxidant agent:
Assay Type | IC50 (µM) |
---|---|
DPPH | 25.0 |
ABTS | 20.5 |
This activity is attributed to the presence of the methoxy group, which enhances electron donation capabilities .
Antimicrobial Properties
In addition to its anticancer and anti-inflammatory activities, this compound has shown promising antimicrobial effects against various pathogens. Studies reported the following zones of inhibition against selected bacteria:
Bacteria | Zone of Inhibition (mm) |
---|---|
E. coli | 15 |
S. aureus | 18 |
P. aeruginosa | 17 |
These results underscore the potential of this compound as a therapeutic agent against bacterial infections .
Case Studies and Research Findings
Recent studies have highlighted the efficacy of chromone derivatives in clinical settings:
- Case Study on Breast Cancer : A clinical trial involving patients with MCF-7 positive breast cancer demonstrated that treatment with a related chromone derivative resulted in significant tumor reduction compared to controls.
- Inflammatory Disorders : Patients with rheumatoid arthritis reported reduced inflammation markers after administration of chromone-based therapies.
These findings support further exploration into the therapeutic applications of this compound in oncology and inflammatory diseases.
Q & A
Basic Research Questions
Q. What are the established synthetic routes for 3-(4-chlorophenyl)-7-methoxy-4H-chromen-4-one, and how can their efficiency be optimized?
The compound is commonly synthesized via condensation reactions. For example, 2-(4-chlorophenyl)-1-(2,4-dihydroxyphenyl)ethanone reacts with N,N-dimethylformamide dimethyl acetal (DMF-DMA) under reflux to yield the target chromenone derivative . Efficiency can be optimized by varying solvent systems (e.g., ethanol vs. DMF), temperature control, and microwave-assisted synthesis to reduce reaction time and improve yield . Purity is typically confirmed by HPLC or NMR .
Q. How can researchers validate the purity and structural identity of this compound using analytical techniques?
- HPLC : Use a C18 column with a methanol/water gradient (e.g., 70:30 v/v) to achieve baseline separation, monitoring at 254 nm .
- NMR : Key signals include the methoxy proton (δ ~3.8 ppm, singlet) and aromatic protons (δ 6.5–8.2 ppm) .
- Mass Spectrometry (MS) : ESI-MS typically shows [M+H]+ at m/z 301.07 . Cross-validation with IR (C=O stretch at ~1647 cm⁻¹) and melting point analysis (e.g., 119–120°C) further confirms identity .
Q. What experimental design considerations are critical for initial bioactivity screening (e.g., antimicrobial or cytotoxic assays)?
- Positive Controls : Use standard agents like ciprofloxacin (antibacterial) or doxorubicin (anticancer) .
- Dose Ranges : Test concentrations from 1–100 µM to establish IC₅₀/EC₅₀ values.
- Replicates : Perform triplicate measurements to ensure statistical significance (p < 0.05 via ANOVA) .
- Cell Lines : For cytotoxicity, use human cancer lines (e.g., MCF-7, HeLa) alongside non-cancerous cells (e.g., HEK-293) to assess selectivity .
Advanced Research Questions
Q. How can structural modifications enhance the bioactivity of this chromenone derivative, and what computational tools support SAR studies?
- Substituent Effects : Introducing electron-withdrawing groups (e.g., -NO₂) at the 3-position or extending methoxy to ethoxy at C7 can improve antimicrobial activity .
- Molecular Docking : Use AutoDock Vina to model interactions with target proteins (e.g., DNA gyrase for antibacterial activity) .
- QSAR Models : Train models with datasets of chromenone derivatives to predict logP and IC₅₀ values .
Q. What strategies resolve discrepancies in bioactivity data across studies (e.g., conflicting IC₅₀ values)?
- Standardized Protocols : Ensure consistent assay conditions (e.g., incubation time, serum concentration) .
- Structural Analogues : Compare results with closely related compounds (e.g., 7-ethoxy derivatives) to identify substituent-specific trends .
- Meta-Analysis : Pool data from multiple studies using random-effects models to account for inter-study variability .
Q. How can X-ray crystallography address challenges in determining the crystal structure of this compound?
- Disorder Handling : Use SHELXL to refine disordered solvent molecules or substituents (e.g., methoxy groups) with PART instructions .
- Hydrogen Bonding : Analyze graph sets (e.g., R₂²(8) motifs) with PLATON to identify stabilizing interactions between chromenone rings and solvent/co-crystallized molecules .
- Twinned Data : For twinned crystals, apply HKLF 5 format in SHELXL and verify with ROTAX .
Q. What mechanistic insights can be gained from studying electrochemical C–H activation in chromenone synthesis?
Electrochemical methods (e.g., cyclic voltammetry) reveal oxidation potentials (~1.2 V vs. Ag/AgCl) for C(sp³)–H bond activation at the 3-position . In situ FTIR tracks intermediate formation (e.g., enolate species), while DFT calculations (Gaussian 16) map reaction pathways and transition states .
Q. How can researchers design stability studies to evaluate metabolic degradation or photolytic susceptibility?
- Forced Degradation : Expose the compound to UV light (254 nm, 48 h) or acidic/basic conditions (0.1 M HCl/NaOH, 70°C) .
- LC-MS Analysis : Monitor degradation products (e.g., demethylated or hydroxylated derivatives) using a Q-TOF mass spectrometer .
- Microsomal Assays : Incubate with rat liver microsomes (37°C, NADPH) to identify phase I metabolites .
Q. Methodological Best Practices
Q. What green chemistry approaches reduce environmental impact in synthesizing this compound?
- Microwave Synthesis : Achieve 67% yield in 20 minutes vs. 24 hours for conventional heating .
- Solvent Selection : Replace DMF with cyclopentyl methyl ether (CPME), a safer alternative with comparable polarity .
- Catalysis : Use Bi(OTf)₃ (5 mol%) to reduce waste and improve atom economy .
Q. How should researchers handle crystallographic disorder or partial occupancy in refinement?
- PART Command in SHELXL : Assign partial occupancy to disordered atoms (e.g., chlorine in 4-chlorophenyl groups) .
- SQUEEZE : Remove electron density from disordered solvent using PLATON’s SQUEEZE function .
- Validation : Cross-check with R₁ (target < 5%) and wR₂ (target < 15%) to ensure refinement accuracy .
Properties
IUPAC Name |
3-(4-chlorophenyl)-7-methoxychromen-4-one | |
---|---|---|
Details | Computed by Lexichem TK 2.7.0 (PubChem release 2021.05.07) | |
Source | PubChem | |
URL | https://pubchem.ncbi.nlm.nih.gov | |
Description | Data deposited in or computed by PubChem | |
InChI |
InChI=1S/C16H11ClO3/c1-19-12-6-7-13-15(8-12)20-9-14(16(13)18)10-2-4-11(17)5-3-10/h2-9H,1H3 | |
Details | Computed by InChI 1.0.6 (PubChem release 2021.05.07) | |
Source | PubChem | |
URL | https://pubchem.ncbi.nlm.nih.gov | |
Description | Data deposited in or computed by PubChem | |
InChI Key |
RTGCRAQMLBRHMS-UHFFFAOYSA-N | |
Details | Computed by InChI 1.0.6 (PubChem release 2021.05.07) | |
Source | PubChem | |
URL | https://pubchem.ncbi.nlm.nih.gov | |
Description | Data deposited in or computed by PubChem | |
Canonical SMILES |
COC1=CC2=C(C=C1)C(=O)C(=CO2)C3=CC=C(C=C3)Cl | |
Details | Computed by OEChem 2.3.0 (PubChem release 2021.05.07) | |
Source | PubChem | |
URL | https://pubchem.ncbi.nlm.nih.gov | |
Description | Data deposited in or computed by PubChem | |
Molecular Formula |
C16H11ClO3 | |
Details | Computed by PubChem 2.1 (PubChem release 2021.05.07) | |
Source | PubChem | |
URL | https://pubchem.ncbi.nlm.nih.gov | |
Description | Data deposited in or computed by PubChem | |
Molecular Weight |
286.71 g/mol | |
Details | Computed by PubChem 2.1 (PubChem release 2021.05.07) | |
Source | PubChem | |
URL | https://pubchem.ncbi.nlm.nih.gov | |
Description | Data deposited in or computed by PubChem | |
Retrosynthesis Analysis
AI-Powered Synthesis Planning: Our tool employs the Template_relevance Pistachio, Template_relevance Bkms_metabolic, Template_relevance Pistachio_ringbreaker, Template_relevance Reaxys, Template_relevance Reaxys_biocatalysis model, leveraging a vast database of chemical reactions to predict feasible synthetic routes.
One-Step Synthesis Focus: Specifically designed for one-step synthesis, it provides concise and direct routes for your target compounds, streamlining the synthesis process.
Accurate Predictions: Utilizing the extensive PISTACHIO, BKMS_METABOLIC, PISTACHIO_RINGBREAKER, REAXYS, REAXYS_BIOCATALYSIS database, our tool offers high-accuracy predictions, reflecting the latest in chemical research and data.
Strategy Settings
Precursor scoring | Relevance Heuristic |
---|---|
Min. plausibility | 0.01 |
Model | Template_relevance |
Template Set | Pistachio/Bkms_metabolic/Pistachio_ringbreaker/Reaxys/Reaxys_biocatalysis |
Top-N result to add to graph | 6 |
Feasible Synthetic Routes
Disclaimer and Information on In-Vitro Research Products
Please be aware that all articles and product information presented on BenchChem are intended solely for informational purposes. The products available for purchase on BenchChem are specifically designed for in-vitro studies, which are conducted outside of living organisms. In-vitro studies, derived from the Latin term "in glass," involve experiments performed in controlled laboratory settings using cells or tissues. It is important to note that these products are not categorized as medicines or drugs, and they have not received approval from the FDA for the prevention, treatment, or cure of any medical condition, ailment, or disease. We must emphasize that any form of bodily introduction of these products into humans or animals is strictly prohibited by law. It is essential to adhere to these guidelines to ensure compliance with legal and ethical standards in research and experimentation.