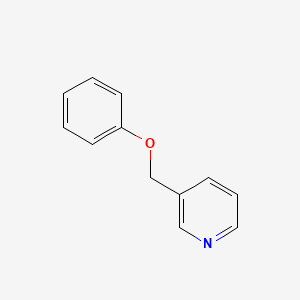
3-(phenoxymethyl)pyridine
Overview
Description
3-(Phenoxymethyl)pyridine is an organic compound with the molecular formula C12H11NO It consists of a pyridine ring substituted at the third position with a phenoxymethyl group
Preparation Methods
Synthetic Routes and Reaction Conditions: The synthesis of 3-(phenoxymethyl)pyridine can be achieved through several methods. One common approach involves the reaction of 3-chloromethylpyridine with phenol in the presence of a base such as potassium carbonate. The reaction typically proceeds under reflux conditions in a suitable solvent like dimethylformamide or acetonitrile.
Industrial Production Methods: On an industrial scale, the production of this compound may involve continuous flow processes to ensure higher yields and purity. The use of catalysts and optimized reaction conditions can further enhance the efficiency of the synthesis.
Chemical Reactions Analysis
Types of Reactions: 3-(Phenoxymethyl)pyridine undergoes various chemical reactions, including:
Oxidation: The compound can be oxidized to form corresponding pyridine N-oxides.
Reduction: Reduction reactions can convert the pyridine ring to piperidine derivatives.
Substitution: Nucleophilic substitution reactions can occur at the phenoxymethyl group, leading to the formation of different derivatives.
Common Reagents and Conditions:
Oxidation: Reagents such as hydrogen peroxide or m-chloroperbenzoic acid are commonly used.
Reduction: Catalytic hydrogenation using palladium on carbon is a typical method.
Substitution: Reagents like sodium hydride or potassium tert-butoxide are often employed.
Major Products:
Oxidation: Pyridine N-oxides.
Reduction: Piperidine derivatives.
Substitution: Various substituted pyridine derivatives.
Scientific Research Applications
3-(Phenoxymethyl)pyridine has diverse applications in scientific research:
Chemistry: It serves as a building block for the synthesis of more complex organic molecules.
Biology: The compound is used in the study of enzyme inhibition and receptor binding.
Industry: It is utilized in the production of agrochemicals and specialty chemicals.
Mechanism of Action
The mechanism of action of 3-(phenoxymethyl)pyridine involves its interaction with specific molecular targets. For instance, in biological systems, it may bind to enzymes or receptors, modulating their activity. The phenoxymethyl group can enhance the compound’s binding affinity and specificity, leading to more effective inhibition or activation of the target molecules.
Comparison with Similar Compounds
- 3-(Phenoxymethyl)-3-oxetanylamine
- 2-(Phenoxymethyl)benzylamine
- 4-(3-Pyridinyl)-2-pyrimidine amine
Uniqueness: 3-(Phenoxymethyl)pyridine is unique due to its specific substitution pattern, which imparts distinct chemical and biological properties. Compared to similar compounds, it may exhibit different reactivity and binding characteristics, making it valuable for targeted applications in research and industry.
Properties
IUPAC Name |
3-(phenoxymethyl)pyridine | |
---|---|---|
Details | Computed by Lexichem TK 2.7.0 (PubChem release 2021.05.07) | |
Source | PubChem | |
URL | https://pubchem.ncbi.nlm.nih.gov | |
Description | Data deposited in or computed by PubChem | |
InChI |
InChI=1S/C12H11NO/c1-2-6-12(7-3-1)14-10-11-5-4-8-13-9-11/h1-9H,10H2 | |
Details | Computed by InChI 1.0.6 (PubChem release 2021.05.07) | |
Source | PubChem | |
URL | https://pubchem.ncbi.nlm.nih.gov | |
Description | Data deposited in or computed by PubChem | |
InChI Key |
QWAMJXOIACKDRQ-UHFFFAOYSA-N | |
Details | Computed by InChI 1.0.6 (PubChem release 2021.05.07) | |
Source | PubChem | |
URL | https://pubchem.ncbi.nlm.nih.gov | |
Description | Data deposited in or computed by PubChem | |
Canonical SMILES |
C1=CC=C(C=C1)OCC2=CN=CC=C2 | |
Details | Computed by OEChem 2.3.0 (PubChem release 2021.05.07) | |
Source | PubChem | |
URL | https://pubchem.ncbi.nlm.nih.gov | |
Description | Data deposited in or computed by PubChem | |
Molecular Formula |
C12H11NO | |
Details | Computed by PubChem 2.1 (PubChem release 2021.05.07) | |
Source | PubChem | |
URL | https://pubchem.ncbi.nlm.nih.gov | |
Description | Data deposited in or computed by PubChem | |
Molecular Weight |
185.22 g/mol | |
Details | Computed by PubChem 2.1 (PubChem release 2021.05.07) | |
Source | PubChem | |
URL | https://pubchem.ncbi.nlm.nih.gov | |
Description | Data deposited in or computed by PubChem | |
Synthesis routes and methods
Procedure details
Retrosynthesis Analysis
AI-Powered Synthesis Planning: Our tool employs the Template_relevance Pistachio, Template_relevance Bkms_metabolic, Template_relevance Pistachio_ringbreaker, Template_relevance Reaxys, Template_relevance Reaxys_biocatalysis model, leveraging a vast database of chemical reactions to predict feasible synthetic routes.
One-Step Synthesis Focus: Specifically designed for one-step synthesis, it provides concise and direct routes for your target compounds, streamlining the synthesis process.
Accurate Predictions: Utilizing the extensive PISTACHIO, BKMS_METABOLIC, PISTACHIO_RINGBREAKER, REAXYS, REAXYS_BIOCATALYSIS database, our tool offers high-accuracy predictions, reflecting the latest in chemical research and data.
Strategy Settings
Precursor scoring | Relevance Heuristic |
---|---|
Min. plausibility | 0.01 |
Model | Template_relevance |
Template Set | Pistachio/Bkms_metabolic/Pistachio_ringbreaker/Reaxys/Reaxys_biocatalysis |
Top-N result to add to graph | 6 |
Feasible Synthetic Routes
Disclaimer and Information on In-Vitro Research Products
Please be aware that all articles and product information presented on BenchChem are intended solely for informational purposes. The products available for purchase on BenchChem are specifically designed for in-vitro studies, which are conducted outside of living organisms. In-vitro studies, derived from the Latin term "in glass," involve experiments performed in controlled laboratory settings using cells or tissues. It is important to note that these products are not categorized as medicines or drugs, and they have not received approval from the FDA for the prevention, treatment, or cure of any medical condition, ailment, or disease. We must emphasize that any form of bodily introduction of these products into humans or animals is strictly prohibited by law. It is essential to adhere to these guidelines to ensure compliance with legal and ethical standards in research and experimentation.