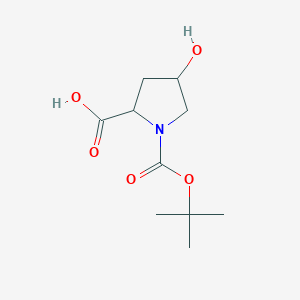
1-(Tert-butoxycarbonyl)-4-hydroxypyrrolidine-2-carboxylic acid
Overview
Description
1-(Tert-butoxycarbonyl)-4-hydroxypyrrolidine-2-carboxylic acid is a compound that features a pyrrolidine ring substituted with a tert-butoxycarbonyl group and a hydroxyl group. This compound is often used in organic synthesis, particularly in the preparation of peptides and other complex molecules. The tert-butoxycarbonyl group serves as a protecting group for amines, making it a valuable tool in synthetic chemistry.
Mechanism of Action
Target of Action
The primary target of 1-[(tert-butoxy)carbonyl]-4-hydroxypyrrolidine-2-carboxylic acid is amines . The compound is used as a protecting group for amines in organic synthesis .
Mode of Action
The compound, also known as the tert-butyloxycarbonyl (BOC) group , can be added to amines under aqueous conditions using di-tert-butyl dicarbonate in the presence of a base such as sodium hydroxide . This results in the protection of the amine group during the synthesis process .
Biochemical Pathways
The BOC group plays a crucial role in the synthesis of complex organic compounds. It protects the amine group from reacting with other reagents during the synthesis process . After the synthesis is complete, the BOC group can be removed with strong acids such as trifluoroacetic acid in dichloromethane, or with HCl in methanol .
Pharmacokinetics
It’s known that the compound is a white solid at room temperature and is soluble in some organic solvents such as ethanol and dimethylformamide .
Result of Action
The primary result of the action of 1-[(tert-butoxy)carbonyl]-4-hydroxypyrrolidine-2-carboxylic acid is the protection of amines during organic synthesis . This allows for the successful synthesis of complex organic compounds without unwanted reactions involving the amine group .
Action Environment
The action of 1-[(tert-butoxy)carbonyl]-4-hydroxypyrrolidine-2-carboxylic acid is influenced by several environmental factors. For instance, the addition of the BOC group to amines requires aqueous conditions and the presence of a base . The removal of the BOC group, on the other hand, requires the presence of strong acids . Therefore, the efficacy and stability of the compound’s action are highly dependent on the specific conditions of the reaction environment .
Preparation Methods
Synthetic Routes and Reaction Conditions: 1-(Tert-butoxycarbonyl)-4-hydroxypyrrolidine-2-carboxylic acid can be synthesized through various methods. One common approach involves the protection of the amine group in pyrrolidine with a tert-butoxycarbonyl group using di-tert-butyl dicarbonate in the presence of a base such as sodium hydroxide . The hydroxyl group can be introduced through subsequent reactions, such as oxidation or substitution reactions.
Industrial Production Methods: Industrial production of this compound typically involves large-scale synthesis using flow microreactor systems. These systems allow for efficient and sustainable synthesis by providing precise control over reaction conditions . The use of flow microreactors also enhances the scalability and reproducibility of the synthesis process.
Chemical Reactions Analysis
Types of Reactions: 1-(Tert-butoxycarbonyl)-4-hydroxypyrrolidine-2-carboxylic acid undergoes various chemical reactions, including:
Oxidation: The hydroxyl group can be oxidized to form a carbonyl group.
Reduction: The carbonyl group can be reduced back to a hydroxyl group.
Substitution: The tert-butoxycarbonyl group can be substituted with other protecting groups or functional groups.
Common Reagents and Conditions:
Oxidation: Common oxidizing agents include potassium permanganate and chromium trioxide.
Reduction: Reducing agents such as lithium aluminum hydride and sodium borohydride are commonly used.
Substitution: Reagents like trifluoroacetic acid and hydrochloric acid are used for deprotection of the tert-butoxycarbonyl group.
Major Products: The major products formed from these reactions depend on the specific conditions and reagents used. For example, oxidation of the hydroxyl group results in the formation of a carbonyl compound, while reduction of the carbonyl group yields a hydroxyl compound.
Scientific Research Applications
1-(Tert-butoxycarbonyl)-4-hydroxypyrrolidine-2-carboxylic acid has numerous applications in scientific research:
Chemistry: It is used as a building block in the synthesis of complex organic molecules and peptides.
Biology: The compound is employed in the study of enzyme mechanisms and protein interactions.
Medicine: It serves as a precursor in the synthesis of pharmaceutical compounds and drug candidates.
Industry: The compound is utilized in the production of fine chemicals and specialty materials.
Comparison with Similar Compounds
- 1-(Tert-butoxycarbonyl)-4-hydroxypyrrolidine-2-carboxylic acid
- 1-(Tert-butoxycarbonyl)-1H-pyrazol-4-yl boronic acid
- tert-Butyloxycarbonyl-protected amino acids
Comparison: this compound is unique due to its specific substitution pattern on the pyrrolidine ring, which imparts distinct chemical properties and reactivity. Compared to other tert-butoxycarbonyl-protected compounds, it offers a balance of stability and reactivity, making it suitable for a wide range of synthetic applications .
Properties
IUPAC Name |
4-hydroxy-1-[(2-methylpropan-2-yl)oxycarbonyl]pyrrolidine-2-carboxylic acid | |
---|---|---|
Details | Computed by LexiChem 2.6.6 (PubChem release 2019.06.18) | |
Source | PubChem | |
URL | https://pubchem.ncbi.nlm.nih.gov | |
Description | Data deposited in or computed by PubChem | |
InChI |
InChI=1S/C10H17NO5/c1-10(2,3)16-9(15)11-5-6(12)4-7(11)8(13)14/h6-7,12H,4-5H2,1-3H3,(H,13,14) | |
Details | Computed by InChI 1.0.5 (PubChem release 2019.06.18) | |
Source | PubChem | |
URL | https://pubchem.ncbi.nlm.nih.gov | |
Description | Data deposited in or computed by PubChem | |
InChI Key |
BENKAPCDIOILGV-UHFFFAOYSA-N | |
Details | Computed by InChI 1.0.5 (PubChem release 2019.06.18) | |
Source | PubChem | |
URL | https://pubchem.ncbi.nlm.nih.gov | |
Description | Data deposited in or computed by PubChem | |
Canonical SMILES |
CC(C)(C)OC(=O)N1CC(CC1C(=O)O)O | |
Details | Computed by OEChem 2.1.5 (PubChem release 2019.06.18) | |
Source | PubChem | |
URL | https://pubchem.ncbi.nlm.nih.gov | |
Description | Data deposited in or computed by PubChem | |
Molecular Formula |
C10H17NO5 | |
Details | Computed by PubChem 2.1 (PubChem release 2019.06.18) | |
Source | PubChem | |
URL | https://pubchem.ncbi.nlm.nih.gov | |
Description | Data deposited in or computed by PubChem | |
DSSTOX Substance ID |
DTXSID40929761 | |
Record name | 1-(tert-Butoxycarbonyl)-4-hydroxyproline | |
Source | EPA DSSTox | |
URL | https://comptox.epa.gov/dashboard/DTXSID40929761 | |
Description | DSSTox provides a high quality public chemistry resource for supporting improved predictive toxicology. | |
Molecular Weight |
231.25 g/mol | |
Details | Computed by PubChem 2.1 (PubChem release 2021.05.07) | |
Source | PubChem | |
URL | https://pubchem.ncbi.nlm.nih.gov | |
Description | Data deposited in or computed by PubChem | |
CAS No. |
13726-69-7 | |
Record name | NSC343720 | |
Source | DTP/NCI | |
URL | https://dtp.cancer.gov/dtpstandard/servlet/dwindex?searchtype=NSC&outputformat=html&searchlist=343720 | |
Description | The NCI Development Therapeutics Program (DTP) provides services and resources to the academic and private-sector research communities worldwide to facilitate the discovery and development of new cancer therapeutic agents. | |
Explanation | Unless otherwise indicated, all text within NCI products is free of copyright and may be reused without our permission. Credit the National Cancer Institute as the source. | |
Record name | 1-(tert-Butoxycarbonyl)-4-hydroxyproline | |
Source | EPA DSSTox | |
URL | https://comptox.epa.gov/dashboard/DTXSID40929761 | |
Description | DSSTox provides a high quality public chemistry resource for supporting improved predictive toxicology. | |
Disclaimer and Information on In-Vitro Research Products
Please be aware that all articles and product information presented on BenchChem are intended solely for informational purposes. The products available for purchase on BenchChem are specifically designed for in-vitro studies, which are conducted outside of living organisms. In-vitro studies, derived from the Latin term "in glass," involve experiments performed in controlled laboratory settings using cells or tissues. It is important to note that these products are not categorized as medicines or drugs, and they have not received approval from the FDA for the prevention, treatment, or cure of any medical condition, ailment, or disease. We must emphasize that any form of bodily introduction of these products into humans or animals is strictly prohibited by law. It is essential to adhere to these guidelines to ensure compliance with legal and ethical standards in research and experimentation.