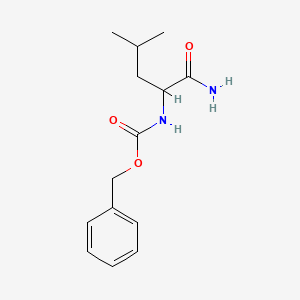
Z-DL-leucine amide
- Click on QUICK INQUIRY to receive a quote from our team of experts.
- With the quality product at a COMPETITIVE price, you can focus more on your research.
Overview
Description
Z-DL-leucine amide: is a synthetic compound with the molecular formula C14H20N2O3 and a molecular weight of 264.32 g/mol . It is a derivative of leucine, an essential amino acid, and is often used in various biochemical and pharmaceutical applications. The compound is characterized by its high purity (≥ 98%) and stability under specific storage conditions (0-8°C) .
Preparation Methods
Synthetic Routes and Reaction Conditions: Z-DL-leucine amide can be synthesized through several methods, including the reaction of leucine with ammonia or amines under controlled conditions. The reaction typically requires a catalyst and is carried out at a temperature range of 129-135°C .
Industrial Production Methods: In industrial settings, the production of this compound often involves large-scale synthesis using automated reactors. The process includes the purification of the final product through crystallization or chromatography to achieve the desired purity levels .
Chemical Reactions Analysis
Types of Reactions: Z-DL-leucine amide undergoes various chemical reactions, including:
Oxidation: The compound can be oxidized to form corresponding oxides.
Reduction: Reduction reactions can convert it into different amine derivatives.
Substitution: It can participate in substitution reactions where the amide group is replaced by other functional groups.
Common Reagents and Conditions:
Oxidation: Common oxidizing agents include hydrogen peroxide and potassium permanganate.
Reduction: Reducing agents such as lithium aluminum hydride are often used.
Substitution: Reagents like halogens and alkylating agents are employed under controlled conditions.
Major Products: The major products formed from these reactions include various derivatives of leucine and amides, which can be further utilized in biochemical applications .
Scientific Research Applications
Chemistry: Z-DL-leucine amide is used as a building block in the synthesis of peptides and other complex molecules. Its stability and reactivity make it a valuable intermediate in organic synthesis .
Biology: In biological research, this compound is used to study protein synthesis and enzyme interactions. It serves as a model compound for understanding the behavior of amino acids in biological systems .
Medicine: Its ability to interact with various biological targets makes it a promising candidate for pharmaceutical research .
Industry: In industrial applications, this compound is used in the production of fine chemicals and as a precursor for other valuable compounds. Its versatility and ease of synthesis make it a preferred choice for large-scale production .
Mechanism of Action
The mechanism of action of Z-DL-leucine amide involves its interaction with specific molecular targets, such as enzymes and receptors. The compound can modulate the activity of these targets by binding to their active sites, thereby influencing various biochemical pathways . For example, it can inhibit certain enzymes involved in amino acid metabolism, leading to altered cellular functions .
Comparison with Similar Compounds
- Z-DL-valine amide
- Z-DL-isoleucine amide
- Z-DL-phenylalanine amide
Comparison: Z-DL-leucine amide is unique due to its specific structural features and reactivity. Compared to similar compounds, it exhibits distinct properties such as higher stability and specific interaction with biological targets . These characteristics make it particularly useful in applications where precise control over chemical reactions is required .
Properties
IUPAC Name |
benzyl N-(1-amino-4-methyl-1-oxopentan-2-yl)carbamate |
Source
|
---|---|---|
Details | Computed by Lexichem TK 2.7.0 (PubChem release 2021.05.07) | |
Source | PubChem | |
URL | https://pubchem.ncbi.nlm.nih.gov | |
Description | Data deposited in or computed by PubChem | |
InChI |
InChI=1S/C14H20N2O3/c1-10(2)8-12(13(15)17)16-14(18)19-9-11-6-4-3-5-7-11/h3-7,10,12H,8-9H2,1-2H3,(H2,15,17)(H,16,18) |
Source
|
Details | Computed by InChI 1.0.6 (PubChem release 2021.05.07) | |
Source | PubChem | |
URL | https://pubchem.ncbi.nlm.nih.gov | |
Description | Data deposited in or computed by PubChem | |
InChI Key |
JZEYMGMOBIYUOV-UHFFFAOYSA-N |
Source
|
Details | Computed by InChI 1.0.6 (PubChem release 2021.05.07) | |
Source | PubChem | |
URL | https://pubchem.ncbi.nlm.nih.gov | |
Description | Data deposited in or computed by PubChem | |
Canonical SMILES |
CC(C)CC(C(=O)N)NC(=O)OCC1=CC=CC=C1 |
Source
|
Details | Computed by OEChem 2.3.0 (PubChem release 2021.05.07) | |
Source | PubChem | |
URL | https://pubchem.ncbi.nlm.nih.gov | |
Description | Data deposited in or computed by PubChem | |
Molecular Formula |
C14H20N2O3 |
Source
|
Details | Computed by PubChem 2.1 (PubChem release 2021.05.07) | |
Source | PubChem | |
URL | https://pubchem.ncbi.nlm.nih.gov | |
Description | Data deposited in or computed by PubChem | |
Molecular Weight |
264.32 g/mol |
Source
|
Details | Computed by PubChem 2.1 (PubChem release 2021.05.07) | |
Source | PubChem | |
URL | https://pubchem.ncbi.nlm.nih.gov | |
Description | Data deposited in or computed by PubChem | |
Disclaimer and Information on In-Vitro Research Products
Please be aware that all articles and product information presented on BenchChem are intended solely for informational purposes. The products available for purchase on BenchChem are specifically designed for in-vitro studies, which are conducted outside of living organisms. In-vitro studies, derived from the Latin term "in glass," involve experiments performed in controlled laboratory settings using cells or tissues. It is important to note that these products are not categorized as medicines or drugs, and they have not received approval from the FDA for the prevention, treatment, or cure of any medical condition, ailment, or disease. We must emphasize that any form of bodily introduction of these products into humans or animals is strictly prohibited by law. It is essential to adhere to these guidelines to ensure compliance with legal and ethical standards in research and experimentation.