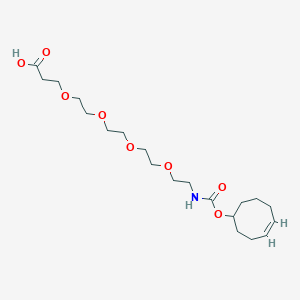
Tco-peg(4)-cooh
Overview
Description
Chemical Structure and Properties TCO-PEG(4)-COOH, also known as (4E)-trans-cyclooctene-tetraethylene glycol-carboxylic acid (CAS: 1802913-21-8), is a trifunctional compound comprising:
- TCO (trans-cyclooctene): A bioorthogonal reactive group that undergoes rapid inverse electron demand Diels-Alder (IEDDA) reactions with tetrazines, enabling applications in live-cell labeling and drug delivery .
- PEG(4): A tetraethylene glycol spacer enhancing water solubility, reducing immunogenicity, and improving biocompatibility .
- Carboxylic acid (-COOH): Facilitates conjugation with amines via carbodiimide chemistry (e.g., EDC/NHS activation) .
Preparation Methods
Synthetic Routes and Reaction Conditions
The synthesis of Tco-peg(4)-cooh typically involves the conjugation of trans-cyclooctene to a polyethylene glycol chain. One common method is the reaction of trans-cyclooctene with a PEG chain that has been functionalized with a carboxylic acid group. This reaction can be facilitated using N-hydroxysuccinimide (NHS) ester chemistry, where the NHS ester of the carboxylic acid reacts with the amine group of trans-cyclooctene .
Industrial Production Methods
Industrial production of this compound involves large-scale synthesis using similar reaction conditions as in laboratory settings. The process is optimized for high yield and purity, often involving purification steps such as high-performance liquid chromatography (HPLC) to ensure the final product meets the required specifications .
Chemical Reactions Analysis
Types of Reactions
Tco-peg(4)-cooh primarily undergoes bioorthogonal reactions, specifically the inverse electron demand Diels–Alder (IEDDA) reaction with tetrazines. This reaction is highly selective and occurs rapidly under physiological conditions .
Common Reagents and Conditions
The IEDDA reaction between this compound and tetrazines is typically carried out in aqueous buffers at room temperature. The reaction does not require a catalyst and proceeds with high efficiency, making it ideal for in vivo applications .
Major Products Formed
The major product formed from the reaction of this compound with tetrazines is a stable covalent adduct, which is often used for labeling biomolecules or for drug delivery applications .
Scientific Research Applications
Bioorthogonal Chemistry
Enhanced Reactivity
TCO-PEG(4)-COOH serves as a crucial component in bioorthogonal reactions, particularly in the context of pretargeting strategies for imaging and therapy. The hydrophilic nature of the PEG linker not only enhances solubility but also preserves the reactivity of TCO. Research indicates that TCO introduced via PEG linkers can significantly enhance functional density without compromising antibody binding activity. For instance, a study demonstrated that using TCO-PEG linkers resulted in over a 5-fold enhancement in reactivity compared to traditional methods .
Dual Bioorthogonal Approach
A novel dual bioorthogonal approach involving TCO-PEG linkers allows for improved coupling with azido-antibodies. This method has shown to increase the functional density of TCOs by more than 10-fold while maintaining high specificity and low background signals during imaging assays . Such enhancements are critical for applications in cancer biomarker imaging, where precise targeting is essential.
Imaging Applications
Fluorescent Imaging
this compound is pivotal in developing advanced imaging techniques. Its incorporation into antibodies allows for high-contrast fluorescent imaging of cancer cells. In one study, confocal imaging revealed that TCO-PEG-modified antibodies produced significantly higher fluorescent signals compared to non-PEGylated counterparts . This capability is vital for visualizing tumor markers and understanding tumor biology.
PET Imaging
In pretargeted positron emission tomography (PET) imaging studies, TCO-PEG derivatives have been evaluated for their efficacy in vivo. The performance of tetrazine (Tz) derivatives conjugated with TCO was assessed, revealing that high IEDDA reactivity correlates with effective blocking effects and improved imaging outcomes. The results indicated that certain Tz-probes achieved blocking efficiencies between 72% and 99%, showcasing the potential of this compound in enhancing PET imaging capabilities .
Therapeutic Applications
Targeted Drug Delivery
The ability of this compound to facilitate selective drug delivery is another significant application. By linking therapeutic agents to TCO moieties, researchers can achieve controlled release mechanisms through bioorthogonal reactions with tetrazines. For example, studies have shown that upon addition of TCO to peptide-fluorophore conjugates, there is a substantial release of the therapeutic agent, indicating the potential for using this system in targeted therapy .
Comparative Analysis of Related Compounds
Compound | Key Features | Application Areas |
---|---|---|
This compound | Enhances solubility and reactivity | Imaging, drug delivery |
DBCO-PEG-TCO | Dual bioorthogonal coupling | Antibody labeling |
Tetrazine Derivatives | High IEDDA reactivity | PET imaging |
Case Studies
Case Study 1: Cancer Biomarker Imaging
A study utilized this compound linked antibodies to target EGFR in A431 cells. The results showed that these modified antibodies produced significantly enhanced fluorescence signals compared to traditional methods, demonstrating the effectiveness of PEG linkers in improving imaging outcomes .
Case Study 2: In Vivo PET Imaging
In another investigation involving pretargeted PET imaging, mice were injected with CC49-TCO followed by administration of fluorine-18-labeled tetrazines. The uptake was quantified using PET/CT scans, revealing that compounds modified with this compound exhibited superior performance in terms of blocking efficiency and tumor uptake compared to controls .
Mechanism of Action
The mechanism of action of Tco-peg(4)-cooh involves its reaction with tetrazines through the IEDDA reaction. The trans-cyclooctene moiety reacts with the tetrazine to form a stable covalent bond, which can be used to link various molecules together. This reaction is highly specific and occurs rapidly, making it suitable for applications in living systems .
Comparison with Similar Compounds
Physical Properties
- Molecular weight: 417.5 g/mol .
- Purity: ≥95% .
- Solubility: Miscible in water and organic solvents (e.g., DMSO, DMF) due to the hydrophilic PEG chain .
- Stability: Stable at -20°C under inert conditions; sensitive to prolonged light exposure .
Comparison with Structurally Similar Compounds
TCO-PEG(4)-NHS (CAS: 1621096-79-4)
Key Differences :
- TCO-PEG(4)-NHS enables direct amine coupling without additional activation reagents, making it ideal for time-sensitive applications . However, its NHS ester is less stable in aqueous media compared to the carboxylic acid group in this compound .
(4E)-TCO-PEG3-COOH (CAS: 2141981-86-2)
Key Differences :
- The shorter PEG chain in TCO-PEG3-COOH reduces steric hindrance, accelerating tetrazine click reactions. However, its lower molecular weight may compromise solubility in complex biological matrices .
TCO-PEG(3)-MAL (CAS: 1609659-01-9)
Property | This compound | TCO-PEG(3)-MAL |
---|---|---|
Reactive Group | Carboxylic acid (-COOH) | Maleimide (-MAL) |
Target | Amines | Thiols (e.g., cysteine residues) |
PEG Units | 4 | 3 |
Applications | Broad bioconjugation | Site-specific antibody labeling |
Key Differences :
- Maleimide in TCO-PEG(3)-MAL enables thiol-specific conjugation, critical for site-directed protein modification. However, maleimides are prone to hydrolysis and thiol exchange in vivo, limiting stability compared to carbodiimide-mediated amide bonds .
Functional Comparison with Non-TCO Analogues
Azido-PEG6-COOH (CAS: N/A)
- Structure : Azide (-N3) + PEG6 + COOH.
- Reactivity : Azide participates in copper-catalyzed alkyne-azide cycloaddition (CuAAC), which is slower than TCO-tetrazine IEDDA .
- Applications : Less suitable for live-cell labeling due to copper toxicity but widely used in surface functionalization .
DBCO-PEG-COOH
- Structure : Dibenzocyclooctyne (DBCO) + PEG + COOH.
- Reactivity : Copper-free click chemistry with azides; slower kinetics than TCO-tetrazine .
- Advantage: No metal catalyst required, making it biocompatible for in vivo use .
Research Findings and Performance Data
- Reaction Rate : this compound exhibits a second-order rate constant of ~1,000 M⁻¹s⁻¹ with methyltetrazine, outperforming DBCO (10–100 M⁻¹s⁻¹) and azides (0.1–1 M⁻¹s⁻¹) .
- In Vivo Stability: PEG(4) extends circulation half-life to >6 hours in murine models, compared to 1–2 hours for non-PEGylated analogues .
- Cytotoxicity: No significant cytotoxicity observed at concentrations ≤100 µM in HEK293 cells .
Biological Activity
TCO-PEG(4)-COOH (trans-cyclooctene polyethylene glycol carboxylic acid) is a significant compound in the field of bioorthogonal chemistry, particularly known for its role in selective labeling and drug delivery applications. This article provides an overview of its biological activity, including its reactivity in bioorthogonal reactions, applications in targeted therapies, and specific case studies that highlight its efficacy.
This compound is characterized by its unique structure, which includes a trans-cyclooctene moiety linked to a polyethylene glycol (PEG) chain. The PEG component enhances solubility and biocompatibility, making it suitable for use in biological systems. The carboxylic acid group allows for further functionalization, enabling conjugation with various biomolecules.
Property | Value |
---|---|
Molecular Weight | 514.57 g/mol |
Chemical Formula | C24H38N2O10 |
Solubility | DMSO, DMF, THF |
Purity | >95% (HPLC) |
Appearance | Colorless to slightly yellow oil |
The primary mechanism by which this compound exerts its biological activity is through the inverse electron demand Diels-Alder (IEDDA) reaction with tetrazine derivatives. This reaction is highly selective and rapid, allowing for efficient labeling of biomolecules in complex biological environments.
Reaction Dynamics
The reaction kinetics of TCO with tetrazines have been extensively studied. For instance, the reaction rate constants can exceed 25,000 Ms per TCO unit when optimized conditions are met . This high reactivity is beneficial for applications requiring rapid and specific labeling of targets within living cells or tissues.
Applications in Targeted Drug Delivery
This compound has been utilized in various biomedical applications, particularly in the development of targeted drug delivery systems. Its ability to form stable conjugates with therapeutic agents allows for controlled release mechanisms that can be triggered by specific stimuli.
Case Study: Tumor Targeting
In a study involving mice models, this compound was conjugated to a radioligand for PET imaging. The results indicated a rapid clearance from circulation and significant accumulation at tumor sites . This demonstrates the potential of this compound in enhancing the targeting efficiency of imaging agents and therapeutic compounds.
Bioorthogonal Chemistry Applications
The use of this compound extends beyond drug delivery; it is also pivotal in bioorthogonal chemistry for labeling proteins and antibodies. The compound's solubility in aqueous buffers facilitates its application in various biological assays.
Table 2: Applications of this compound
Application | Description |
---|---|
Protein Labeling | Enables selective tagging of proteins for studies |
Antibody Conjugation | Enhances specificity in targeting cancer cells |
Imaging Agent Development | Used in PET imaging for real-time monitoring of tumors |
Research Findings
Recent studies have highlighted the effectiveness of this compound in various experimental setups:
- Selective Activation : A study demonstrated that prodrugs activated via metabolic processes showed improved efficacy when combined with tetrazine ligation strategies involving TCO .
- Enzyme Activity Retention : Research indicated that enzymes modified with TCO-PEG retained their catalytic activity comparable to their native forms, suggesting minimal disruption from the conjugation process .
- Biodistribution Studies : Biodistribution analyses revealed that TCO-conjugated compounds exhibited rapid renal clearance, which is advantageous for reducing systemic toxicity while maintaining therapeutic efficacy .
Q & A
Basic Research Questions
Q. What are the primary chemical properties of TCO-PEG(4)-COOH, and how do they influence its reactivity in conjugation reactions?
- Answer : this compound is a heterobifunctional linker with a trans-cyclooctene (TCO) group for bioorthogonal click chemistry (e.g., tetrazine ligation) and a carboxylate (-COOH) group for covalent coupling to amines. Key properties include:
- Hydrophilicity : PEG(4) spacer enhances solubility in aqueous buffers, critical for bioconjugation .
- pH sensitivity : Carboxylate group reactivity depends on pH (optimal activation at pH 4.5–5.5 via EDC/NHS chemistry) .
- Stability : TCO’s ring strain drives fast ligation kinetics but may degrade under prolonged light/heat exposure. Characterize via HPLC or NMR to confirm integrity .
Q. Which characterization techniques are essential for verifying this compound purity and functional group integrity?
- Answer :
Advanced Research Questions
Q. How can researchers optimize this compound conjugation efficiency while minimizing side reactions (e.g., hydrolysis or PEG oxidation)?
- Answer :
- Controlled reaction conditions : Use anhydrous solvents (e.g., DMF) and inert atmospheres to reduce hydrolysis. Monitor pH (5.0–6.0) during carboxylate activation .
- Molar ratio optimization : Conduct pilot reactions with varying this compound:target molecule ratios (e.g., 1:1 to 5:1) to balance yield and excess reagent removal .
- Stabilizers : Add antioxidants (e.g., ascorbic acid) to prevent PEG oxidation under light. Validate via MALDI-TOF .
Q. What experimental strategies address contradictions in reported this compound conjugation efficiencies across studies?
- Answer : Systematic analysis using the PICO framework (Population: this compound; Intervention: reaction parameters; Comparison: conflicting studies; Outcome: efficiency metrics):
- Variable isolation : Test individual factors (e.g., temperature, solvent purity, ligand density) in controlled experiments .
- Meta-analysis : Aggregate data from literature (exclude non-peer-reviewed sources like BenchChem) and apply statistical models (e.g., ANOVA) to identify outliers .
- Reproducibility checks : Replicate high-discrepancy studies with standardized protocols (e.g., fixed NHS:EDC ratios) .
Q. Methodological Challenges
Q. How should researchers design studies to evaluate this compound’s biocompatibility in vivo while accounting for PEG immunogenicity?
- Answer :
- PEO framework : Define Population (animal model), Exposure (PEG(4) length/dose), Outcome (immune response metrics like cytokine levels) .
- Controls : Include PEG-free analogues and commercial PEGylated controls (e.g., PEG-IFNα) to isolate immunogenicity effects .
- Longitudinal tracking : Use ELISA or flow cytometry to monitor anti-PEG antibodies over time .
Q. What are the best practices for synthesizing this compound derivatives with tailored functionalities (e.g., fluorescent labeling)?
- Answer :
- Modular design : Introduce functional handles (e.g., azide, DBCO) during PEG synthesis via orthogonal protection strategies .
- Stepwise validation : Confirm intermediate purity after each modification (e.g., TLC for azide incorporation) before proceeding .
- Activity assays : Test final conjugates in model reactions (e.g., tetrazine ligation kinetics via UV-Vis) to ensure retained functionality .
Q. Data Analysis & Interpretation
Q. How can researchers resolve discrepancies in this compound stability data under varying storage conditions?
- Answer : Apply the FINER criteria (Feasible, Interesting, Novel, Ethical, Relevant):
- Accelerated stability studies : Store samples at 4°C, 25°C, and 40°C with humidity control. Analyze degradation via LC-MS at intervals (e.g., 0, 7, 30 days) .
- Multivariate regression : Correlative analysis of degradation rates with environmental factors (e.g., O₂ levels) .
- Peer benchmarking : Compare results with published stability data from authoritative journals (exclude non-indexed sources) .
Q. Tables for Reference
Table 1 : Common Challenges in this compound Synthesis
Table 2 : Frameworks for Structuring Research Questions
Properties
IUPAC Name |
3-[2-[2-[2-[2-[[(4E)-cyclooct-4-en-1-yl]oxycarbonylamino]ethoxy]ethoxy]ethoxy]ethoxy]propanoic acid | |
---|---|---|
Details | Computed by LexiChem 2.6.6 (PubChem release 2019.06.18) | |
Source | PubChem | |
URL | https://pubchem.ncbi.nlm.nih.gov | |
Description | Data deposited in or computed by PubChem | |
InChI |
InChI=1S/C20H35NO8/c22-19(23)8-10-25-12-14-27-16-17-28-15-13-26-11-9-21-20(24)29-18-6-4-2-1-3-5-7-18/h1-2,18H,3-17H2,(H,21,24)(H,22,23)/b2-1+ | |
Details | Computed by InChI 1.0.5 (PubChem release 2019.06.18) | |
Source | PubChem | |
URL | https://pubchem.ncbi.nlm.nih.gov | |
Description | Data deposited in or computed by PubChem | |
InChI Key |
PSNKSDUNMXNXDE-OWOJBTEDSA-N | |
Details | Computed by InChI 1.0.5 (PubChem release 2019.06.18) | |
Source | PubChem | |
URL | https://pubchem.ncbi.nlm.nih.gov | |
Description | Data deposited in or computed by PubChem | |
Canonical SMILES |
C1CC=CCCC(C1)OC(=O)NCCOCCOCCOCCOCCC(=O)O | |
Details | Computed by OEChem 2.1.5 (PubChem release 2019.06.18) | |
Source | PubChem | |
URL | https://pubchem.ncbi.nlm.nih.gov | |
Description | Data deposited in or computed by PubChem | |
Isomeric SMILES |
C1C/C=C/CCC(C1)OC(=O)NCCOCCOCCOCCOCCC(=O)O | |
Details | Computed by OEChem 2.1.5 (PubChem release 2019.06.18) | |
Source | PubChem | |
URL | https://pubchem.ncbi.nlm.nih.gov | |
Description | Data deposited in or computed by PubChem | |
Molecular Formula |
C20H35NO8 | |
Details | Computed by PubChem 2.1 (PubChem release 2019.06.18) | |
Source | PubChem | |
URL | https://pubchem.ncbi.nlm.nih.gov | |
Description | Data deposited in or computed by PubChem | |
Molecular Weight |
417.5 g/mol | |
Details | Computed by PubChem 2.1 (PubChem release 2021.05.07) | |
Source | PubChem | |
URL | https://pubchem.ncbi.nlm.nih.gov | |
Description | Data deposited in or computed by PubChem | |
Retrosynthesis Analysis
AI-Powered Synthesis Planning: Our tool employs the Template_relevance Pistachio, Template_relevance Bkms_metabolic, Template_relevance Pistachio_ringbreaker, Template_relevance Reaxys, Template_relevance Reaxys_biocatalysis model, leveraging a vast database of chemical reactions to predict feasible synthetic routes.
One-Step Synthesis Focus: Specifically designed for one-step synthesis, it provides concise and direct routes for your target compounds, streamlining the synthesis process.
Accurate Predictions: Utilizing the extensive PISTACHIO, BKMS_METABOLIC, PISTACHIO_RINGBREAKER, REAXYS, REAXYS_BIOCATALYSIS database, our tool offers high-accuracy predictions, reflecting the latest in chemical research and data.
Strategy Settings
Precursor scoring | Relevance Heuristic |
---|---|
Min. plausibility | 0.01 |
Model | Template_relevance |
Template Set | Pistachio/Bkms_metabolic/Pistachio_ringbreaker/Reaxys/Reaxys_biocatalysis |
Top-N result to add to graph | 6 |
Feasible Synthetic Routes
Disclaimer and Information on In-Vitro Research Products
Please be aware that all articles and product information presented on BenchChem are intended solely for informational purposes. The products available for purchase on BenchChem are specifically designed for in-vitro studies, which are conducted outside of living organisms. In-vitro studies, derived from the Latin term "in glass," involve experiments performed in controlled laboratory settings using cells or tissues. It is important to note that these products are not categorized as medicines or drugs, and they have not received approval from the FDA for the prevention, treatment, or cure of any medical condition, ailment, or disease. We must emphasize that any form of bodily introduction of these products into humans or animals is strictly prohibited by law. It is essential to adhere to these guidelines to ensure compliance with legal and ethical standards in research and experimentation.