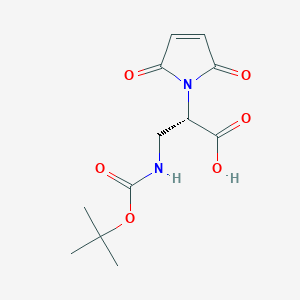
Mal-L-PA-NH-Boc
Overview
Description
Mal-L-PA-NH-Boc, also known as (S)-MALEOYL-DPR (BOC)-OH·DCHA, is a noncleavable antibody-drug conjugate (ADC) linker. This compound is primarily used in the synthesis of antibody-drug conjugates, which are a class of targeted cancer therapies. The compound is characterized by its high purity and stability, making it a valuable tool in the development of therapeutic agents .
Mechanism of Action
Target of Action
The primary target of Mal-L-PA-NH-Boc, also known as Mal-L-Dap(Boc)-OH, is the synthesis of antibody-drug conjugates (ADCs) . ADCs are a class of biopharmaceutical drugs designed as a targeted therapy for treating cancer. They are complex molecules composed of an antibody linked to a biologically active cytotoxic (anti-cancer) payload or drug .
Mode of Action
This compound acts as a non-cleavable linker in the synthesis of ADCs . The linker’s role is to stably attach the cytotoxic drug to the antibody and to release the drug once the ADC has bound to the target cell . As a non-cleavable linker, this compound remains attached to the drug even after the ADC has been internalized by the target cell .
Biochemical Pathways
The biochemical pathways affected by this compound are those involved in the action of ADCs . Once the ADC binds to the target cell, it is internalized and the cytotoxic drug is released, leading to cell death . The exact pathways affected would depend on the specific cytotoxic drug used in the ADC.
Pharmacokinetics
The pharmacokinetics of this compound would be largely determined by the properties of the ADC it is part of . Factors such as absorption, distribution, metabolism, and excretion (ADME) would be influenced by the characteristics of the antibody, the linker, and the cytotoxic drug .
Result of Action
The result of the action of this compound is the successful delivery of the cytotoxic drug to the target cancer cells . This leads to the death of the cancer cells while minimizing damage to healthy cells .
Action Environment
The action environment can significantly influence the efficacy and stability of this compound and the ADCs it helps to create . Factors such as pH, temperature, and the presence of other biological molecules can affect the stability of the ADC and its ability to bind to target cells .
Biochemical Analysis
Biochemical Properties
Mal-L-PA-NH-Boc is primarily used as a linker in the synthesis of antibody-drug conjugates. In biochemical reactions, this compound interacts with various enzymes and proteins involved in the conjugation process. The compound forms stable covalent bonds with the amino groups of lysine residues on antibodies, ensuring that the cytotoxic drug remains attached to the antibody until it reaches the target cancer cell . This interaction is crucial for the stability and efficacy of the ADCs, as it prevents premature release of the drug and ensures targeted delivery.
Cellular Effects
This compound influences various cellular processes by enabling the targeted delivery of cytotoxic drugs to cancer cells. Once the ADCs reach the cancer cells, the cytotoxic drug is released, leading to cell death. This targeted approach minimizes damage to healthy cells and reduces the side effects associated with traditional chemotherapy . This compound, therefore, plays a significant role in enhancing the efficacy and safety of cancer treatments.
Molecular Mechanism
The molecular mechanism of this compound involves its role as a non-cleavable linker in ADCs. The compound forms a stable covalent bond with the antibody, ensuring that the cytotoxic drug remains attached until it reaches the target cancer cell . Upon binding to the cancer cell, the ADC is internalized, and the cytotoxic drug is released, leading to cell death. This mechanism ensures that the drug is delivered specifically to cancer cells, minimizing damage to healthy cells.
Temporal Effects in Laboratory Settings
In laboratory settings, the effects of this compound have been observed to change over time. The compound is stable under various storage conditions, with a shelf life of up to three years when stored as a powder at -20°C . In solution, this compound remains stable for up to six months at -80°C and for one month at -20°C . Long-term studies have shown that the compound maintains its efficacy and stability, making it a reliable component in the synthesis of ADCs.
Dosage Effects in Animal Models
The effects of this compound vary with different dosages in animal models. Studies have shown that the compound is effective at facilitating the targeted delivery of cytotoxic drugs at various dosages . At high doses, there may be toxic or adverse effects, highlighting the importance of optimizing the dosage to achieve the desired therapeutic effect while minimizing side effects .
Metabolic Pathways
This compound is involved in the metabolic pathways associated with the synthesis of ADCs. The compound interacts with enzymes and cofactors involved in the conjugation process, ensuring the stable attachment of the cytotoxic drug to the antibody . This interaction is crucial for the efficacy of the ADCs, as it ensures that the drug is delivered specifically to the target cancer cells.
Transport and Distribution
This compound is transported and distributed within cells and tissues as part of the ADCs. The compound interacts with transporters and binding proteins that facilitate its movement within the body . This interaction ensures that the ADCs reach the target cancer cells, where the cytotoxic drug is released to exert its therapeutic effect.
Subcellular Localization
The subcellular localization of this compound is primarily within the lysosomes of cancer cells. Upon internalization of the ADCs, the compound directs the cytotoxic drug to the lysosomes, where it is released to induce cell death . This targeted approach ensures that the drug is delivered specifically to the cancer cells, minimizing damage to healthy cells.
Preparation Methods
Synthetic Routes and Reaction Conditions
The synthesis of Mal-L-PA-NH-Boc involves several steps, typically starting with the preparation of the maleimide derivative. The maleimide group is introduced through a reaction with maleic anhydride, followed by the protection of the amine group with a tert-butyloxycarbonyl (Boc) group. The final product is obtained through purification processes such as crystallization or chromatography .
Industrial Production Methods
Industrial production of this compound follows similar synthetic routes but on a larger scale. The process involves the use of automated reactors and purification systems to ensure consistency and high yield. Quality control measures, including high-performance liquid chromatography (HPLC) and nuclear magnetic resonance (NMR) spectroscopy, are employed to verify the purity and structure of the compound .
Chemical Reactions Analysis
Types of Reactions
Mal-L-PA-NH-Boc undergoes various chemical reactions, including:
Substitution Reactions: The maleimide group can react with thiol groups to form stable thioether bonds.
Hydrolysis: The Boc protecting group can be removed under acidic conditions to expose the amine group.
Common Reagents and Conditions
Substitution Reactions: Typically involve thiol-containing compounds under mild conditions.
Hydrolysis: Performed using acids such as trifluoroacetic acid (TFA) at room temperature.
Major Products
Thioether Bonds: Formed during substitution reactions with thiol groups.
Free Amine: Obtained after the removal of the Boc protecting group.
Scientific Research Applications
Mal-L-PA-NH-Boc is widely used in scientific research, particularly in the development of antibody-drug conjugates (ADCs). These conjugates are designed to deliver cytotoxic drugs specifically to cancer cells, minimizing damage to healthy tissues. The noncleavable nature of this compound ensures that the drug remains attached to the antibody until it reaches the target cell, enhancing the specificity and efficacy of the treatment .
In addition to its use in ADCs, this compound is also employed in the synthesis of other bioconjugates and in various chemical biology applications. Its stability and reactivity make it a versatile tool for researchers in the fields of chemistry, biology, and medicine .
Comparison with Similar Compounds
Similar Compounds
Succinic Anhydride: Another noncleavable ADC linker used in similar applications.
EMCS (6-Maleimidohexanoic Acid N-Hydroxysuccinimide Ester): A heterobifunctional crosslinking agent used in the preparation of hapten conjugates and enzyme immune conjugates.
Fmoc-8-Amino-3,6-Dioxaoctanoic Acid: A cleavable ADC linker used in the synthesis of antibody-drug conjugates.
Uniqueness
Mal-L-PA-NH-Boc is unique due to its noncleavable nature, which ensures that the drug remains attached to the antibody until it reaches the target cell. This property enhances the specificity and efficacy of ADCs, making this compound a valuable tool in targeted cancer therapy .
Properties
IUPAC Name |
(2S)-2-(2,5-dioxopyrrol-1-yl)-3-[(2-methylpropan-2-yl)oxycarbonylamino]propanoic acid | |
---|---|---|
Details | Computed by LexiChem 2.6.6 (PubChem release 2019.06.18) | |
Source | PubChem | |
URL | https://pubchem.ncbi.nlm.nih.gov | |
Description | Data deposited in or computed by PubChem | |
InChI |
InChI=1S/C12H16N2O6/c1-12(2,3)20-11(19)13-6-7(10(17)18)14-8(15)4-5-9(14)16/h4-5,7H,6H2,1-3H3,(H,13,19)(H,17,18)/t7-/m0/s1 | |
Details | Computed by InChI 1.0.5 (PubChem release 2019.06.18) | |
Source | PubChem | |
URL | https://pubchem.ncbi.nlm.nih.gov | |
Description | Data deposited in or computed by PubChem | |
InChI Key |
QNSOCOXQLKMHCV-ZETCQYMHSA-N | |
Details | Computed by InChI 1.0.5 (PubChem release 2019.06.18) | |
Source | PubChem | |
URL | https://pubchem.ncbi.nlm.nih.gov | |
Description | Data deposited in or computed by PubChem | |
Canonical SMILES |
CC(C)(C)OC(=O)NCC(C(=O)O)N1C(=O)C=CC1=O | |
Details | Computed by OEChem 2.1.5 (PubChem release 2019.06.18) | |
Source | PubChem | |
URL | https://pubchem.ncbi.nlm.nih.gov | |
Description | Data deposited in or computed by PubChem | |
Isomeric SMILES |
CC(C)(C)OC(=O)NC[C@@H](C(=O)O)N1C(=O)C=CC1=O | |
Details | Computed by OEChem 2.1.5 (PubChem release 2019.06.18) | |
Source | PubChem | |
URL | https://pubchem.ncbi.nlm.nih.gov | |
Description | Data deposited in or computed by PubChem | |
Molecular Formula |
C12H16N2O6 | |
Details | Computed by PubChem 2.1 (PubChem release 2019.06.18) | |
Source | PubChem | |
URL | https://pubchem.ncbi.nlm.nih.gov | |
Description | Data deposited in or computed by PubChem | |
Molecular Weight |
284.26 g/mol | |
Details | Computed by PubChem 2.1 (PubChem release 2021.05.07) | |
Source | PubChem | |
URL | https://pubchem.ncbi.nlm.nih.gov | |
Description | Data deposited in or computed by PubChem | |
Retrosynthesis Analysis
AI-Powered Synthesis Planning: Our tool employs the Template_relevance Pistachio, Template_relevance Bkms_metabolic, Template_relevance Pistachio_ringbreaker, Template_relevance Reaxys, Template_relevance Reaxys_biocatalysis model, leveraging a vast database of chemical reactions to predict feasible synthetic routes.
One-Step Synthesis Focus: Specifically designed for one-step synthesis, it provides concise and direct routes for your target compounds, streamlining the synthesis process.
Accurate Predictions: Utilizing the extensive PISTACHIO, BKMS_METABOLIC, PISTACHIO_RINGBREAKER, REAXYS, REAXYS_BIOCATALYSIS database, our tool offers high-accuracy predictions, reflecting the latest in chemical research and data.
Strategy Settings
Precursor scoring | Relevance Heuristic |
---|---|
Min. plausibility | 0.01 |
Model | Template_relevance |
Template Set | Pistachio/Bkms_metabolic/Pistachio_ringbreaker/Reaxys/Reaxys_biocatalysis |
Top-N result to add to graph | 6 |
Feasible Synthetic Routes
Disclaimer and Information on In-Vitro Research Products
Please be aware that all articles and product information presented on BenchChem are intended solely for informational purposes. The products available for purchase on BenchChem are specifically designed for in-vitro studies, which are conducted outside of living organisms. In-vitro studies, derived from the Latin term "in glass," involve experiments performed in controlled laboratory settings using cells or tissues. It is important to note that these products are not categorized as medicines or drugs, and they have not received approval from the FDA for the prevention, treatment, or cure of any medical condition, ailment, or disease. We must emphasize that any form of bodily introduction of these products into humans or animals is strictly prohibited by law. It is essential to adhere to these guidelines to ensure compliance with legal and ethical standards in research and experimentation.