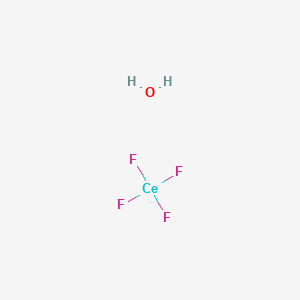
Cerium(IV) fluoride hydrate
Overview
Description
Cerium(IV) fluoride hydrate (CeF₄·xH₂O) is a water-insoluble cerium compound used in oxygen-sensitive applications such as metal production, glass polishing, and ceramics . Its structure likely involves Ce⁴⁺ ions coordinated with fluoride ligands and water molecules, forming a stable lattice. Commercial preparations emphasize its role in scavenging trace elements like lead and antimony during steel manufacturing .
Preparation Methods
Cerium(IV) fluoride hydrate can be synthesized through several methods:
Fluorination of Cerium(III) Fluoride or Cerium Dioxide: This method involves reacting cerium(III) fluoride or cerium dioxide with fluorine gas at 500°C.
Reaction with Hydrofluoric Acid: The hydrated form of cerium(IV) fluoride can be produced by reacting 40% hydrofluoric acid with cerium(IV) sulfate solution at 90°C.
Precipitation Method: By precipitating from an aqueous solution of cerium(IV) sulfate with 40% hydrofluoric acid, a nanosized powder of this compound can be obtained.
Chemical Reactions Analysis
Cerium(IV) fluoride hydrate undergoes various types of chemical reactions:
Oxidation: As a strong oxidant, this compound can oxidize a variety of organic and inorganic compounds.
Reduction: It can be reduced to cerium(III) fluoride under certain conditions.
Substitution: Cerium(IV) fluoride can react with dimethyl sulfoxide (DMSO) to form coordination complexes such as [CeF₄(DMSO)₂].
Common reagents used in these reactions include hydrofluoric acid, sulfuric acid, and various organic solvents. Major products formed from these reactions include cerium(III) fluoride and various coordination complexes .
Scientific Research Applications
Chemical Properties and Characteristics
- Molecular Formula : CeF- xHO
- Molecular Weight : Approximately 234.13 g/mol
- Appearance : White powder
- Melting Point : 100°C
Cerium(IV) fluoride hydrate is characterized by its water-insoluble nature, making it suitable for various applications where moisture sensitivity is critical.
Catalytic Applications
This compound has been demonstrated to act as an effective catalyst in organic synthesis. For instance, it plays a crucial role in the Hantzsch reaction, facilitating the formation of dihydropyridines, which are important intermediates in pharmaceuticals.
Environmental Remediation
A significant study focused on the synthesis of cerium(IV)-incorporated hydrous iron(III) oxide (CIHFO), which utilizes this compound for fluoride ion adsorption from aqueous solutions. The optimized CIHFO demonstrated superior fluoride removal capabilities at neutral pH, with maximum adsorption observed under controlled conditions (temperature and pH adjustments) .
- Adsorption Capacity : The CIHFO material achieved a maximum fluoride adsorption capacity of 24.8 mg/g at optimal conditions.
Synthesis of Advanced Materials
This compound is integral to synthesizing advanced materials such as sodium-rare earth silicate oxide-fluoride apatites. These materials are essential for various applications, including phosphors and solid-state ion conductors used in batteries and fuel cells .
Research Findings
Recent research highlights the hydrothermal transformations of hydrated cerium(IV) fluoride, leading to new crystalline structures with potential applications in catalysis and materials science. For example, a study revealed the formation of a new cerium fluoride hydrate (CeF- HO), which exhibits unique properties that could enhance its utility in various chemical processes .
Mechanism of Action
The mechanism of action of cerium(IV) fluoride hydrate involves its strong oxidizing properties. It can accept electrons from other substances, thereby oxidizing them. This process often involves the formation of intermediate complexes, which play a crucial role in the overall reaction mechanism .
Comparison with Similar Compounds
Comparison with Similar Cerium Compounds
Solubility and Structural Properties
Table 1: Solubility and Structural Characteristics
Key Observations :
- Ce(IV) compounds (e.g., CeO₂, CeF₄·xH₂O) are generally less soluble than Ce(III) analogs (e.g., CeCl₃, CeF₃) due to stronger ionic bonding in higher oxidation states .
- Coordination numbers vary: Ce(IV) in fluoride complexes adopts 8-fold coordination (e.g., CeF₈⁴⁻), while Ce(IV) oxide has 6-fold octahedral geometry .
Antioxidant and Catalytic Activity
Table 2: Antioxidant Performance of Cerium-Based Nanoparticles
Key Observations :
- CeO₂ nanoparticles outperform CeF₃ due to ultra-small size (2–4 nm) and higher surface Ce³⁺/Ce⁴⁺ redox sites .
- Ce(IV) fluoride hydrate, when formed in situ from Ce⁴⁺ and F⁻, exhibits antioxidant activity comparable to CeO₂, suggesting nanoparticle-mediated protection .
Table 3: Application-Specific Performance
Key Observations :
- CeO₂’s catalytic versatility contrasts with Ce(IV) sulfate’s niche role in redox reactions .
Biological Activity
Cerium(IV) fluoride hydrate (CeF·nHO) is a compound of interest due to its unique properties and potential applications in various biological contexts. This article reviews the biological activity of this compound, including its synthesis, structural characteristics, and implications for biomedical applications.
Synthesis and Structural Characteristics
This compound can be synthesized through hydrothermal methods, which involve heating cerium oxide in the presence of hydrofluoric acid. Studies have shown that the compound exhibits a monoclinic crystal structure, which is isostructural to other metal fluorides like uranyl fluoride hydrates . The synthesis process can yield different hydration states, and the specific conditions can significantly influence the resulting crystal structure and properties.
Table 1: Properties of this compound
Property | Value |
---|---|
Chemical Formula | CeF·nHO |
Crystal System | Monoclinic |
Solubility | Soluble in acidic solutions |
Toxicity | Low toxicity in normal cells |
Reactivity | Reacts with strong acids |
Biological Activity
Research has indicated that this compound possesses several biological activities that make it suitable for potential applications in medicine and biotechnology.
Antioxidant Properties
Cerium-based compounds have been studied for their antioxidant properties. For instance, cerium fluoride nanoparticles (CeF) have demonstrated significant protection against oxidative stress in various cell lines. These nanoparticles can scavenge free radicals, thereby reducing cellular damage caused by oxidative stress .
Radioprotective Effects
This compound has shown promise as a radioprotective agent. Studies indicate that cerium fluoride nanoparticles can enhance the radiosensitivity of tumor cells while protecting normal cells from radiation damage. This dual action makes them potential candidates for use in radiotherapy where selective targeting of cancer cells is crucial .
Case Study: Radiosensitization in Cancer Therapy
A notable case study involved the application of cerium fluoride nanoparticles functionalized with flavin mononucleotide (FMN). These nanoparticles were tested for their radiosensitizing effects on MCF-7 breast cancer cells. The results showed a significant increase in radiosensitivity (1.9 times) when exposed to X-ray irradiation at doses between 3 to 6 Gy. In contrast, normal cells maintained high clonogenic activity, highlighting the selective nature of cerium fluoride's biological activity .
The biological mechanisms underlying the effects of this compound are multifaceted:
- Reactive Oxygen Species (ROS) Generation : Upon exposure to radiation, cerium fluoride can generate ROS, which are known to induce apoptosis in cancer cells.
- Antioxidant Defense : The compound's ability to scavenge free radicals contributes to its protective effects on normal cells.
- Metal Ion Interaction : Cerium ions can interact with various biological molecules, potentially influencing cellular signaling pathways .
Q & A
Basic Research Questions
Q. What are the critical physical and chemical properties of Cerium(IV) fluoride hydrate (CeF₄·XH₂O) relevant to experimental design?
CeF₄·XH₂O is a hygroscopic white powder with a decomposition temperature of 650°C and dehydration at 100°C . Key properties include:
- Hygroscopicity : Requires storage in airtight containers under anhydrous conditions to prevent moisture absorption .
- Thermal Stability : Dehydration occurs gradually at 100°C, while decomposition at 650°C necessitates controlled heating in inert atmospheres for high-temperature applications .
- Water Insolubility : Makes it suitable for oxygen-sensitive processes, such as metallurgical applications . Methodological Note : Use thermogravimetric analysis (TGA) to monitor mass loss during dehydration and decomposition .
Q. How can researchers determine the hydration state (X) in CeF₄·XH₂O?
The hydration level is best quantified via TGA by heating the sample from 25°C to 200°C at 5°C/min under nitrogen. The mass loss corresponds to water content. Complementary techniques include:
- Karl Fischer Titration : For precise water quantification in solid samples .
- X-ray Diffraction (XRD) : To confirm crystallographic changes after dehydration .
Q. What safety protocols are essential when handling CeF₄·XH₂O in the laboratory?
- Respiratory Protection : Use N95 masks or fume hoods to avoid inhalation of fine particles, which may irritate airways .
- Thermal Decomposition Mitigation : Avoid heating above 100°C in open air; use inert atmospheres to prevent hazardous fluoride gas release .
- Storage : Keep in sealed containers with desiccants to limit hygroscopic degradation .
Advanced Research Questions
Q. How do competing anions (e.g., NO₃⁻, SO₄²⁻) influence the stability and redox behavior of CeF₄·XH₂O in aqueous systems?
Competing anions can induce redox reactions or complexation:
- Nitrate Ions : May oxidize Ce(III) impurities to Ce(IV), altering stoichiometry .
- Sulfate Ions : Can form stable Ce(IV)-sulfato complexes, reducing CeF₄’s oxidative capacity . Methodological Approach :
- Use UV-Vis spectroscopy to track Ce(IV)/Ce(III) transitions under varying anion concentrations .
- Employ cyclic voltammetry to map redox potentials in acidic media with different electrolytes .
Q. What analytical challenges arise in quantifying cerium speciation in CeF₄·XH₂O, and how can they be resolved?
Challenges include:
- Spectroscopic Interference : Fluoride ligands may obscure Ce(IV) absorbance peaks in UV-Vis .
- Redox Instability : Ce(IV) can reduce to Ce(III) during sample preparation, leading to data discrepancies . Solutions :
- X-ray Photoelectron Spectroscopy (XPS) : Directly quantify Ce oxidation states .
- Controlled Atmosphere Sampling : Perform analyses in gloveboxes to prevent air-induced redox shifts .
Q. How does the hydration state (X) impact CeF₄·XH₂O’s catalytic performance in oxidation reactions?
- Low Hydration (X < 1) : Enhances Lewis acidity, improving catalytic activity in organic oxidations .
- High Hydration (X > 1) : Increases hydrophilicity, favoring aqueous-phase reactions but reducing thermal stability . Experimental Design :
- Synthesize CeF₄ with controlled X via vacuum dehydration at 100°C .
- Test catalytic efficiency in model reactions (e.g., alcohol oxidation) using gas chromatography to monitor product yields .
Q. Data Contradictions and Resolution
Q. Conflicting reports exist on CeF₄·XH₂O’s solubility in fluoride salt mixtures. How can researchers reconcile these discrepancies?
- Issue : Early studies reported solubility in NaF-ZrF₄ melts, while later work noted insolubility .
- Resolution : Variability arises from differences in melt composition and temperature. Methodology :
- Replicate experiments using 141Ce tracer techniques to track dissolution .
- Characterize post-experiment residues via scanning electron microscopy (SEM) to identify undissolved phases .
Properties
IUPAC Name |
tetrafluorocerium;hydrate | |
---|---|---|
Details | Computed by LexiChem 2.6.6 (PubChem release 2019.06.18) | |
Source | PubChem | |
URL | https://pubchem.ncbi.nlm.nih.gov | |
Description | Data deposited in or computed by PubChem | |
InChI |
InChI=1S/Ce.4FH.H2O/h;4*1H;1H2/q+4;;;;;/p-4 | |
Details | Computed by InChI 1.0.5 (PubChem release 2019.06.18) | |
Source | PubChem | |
URL | https://pubchem.ncbi.nlm.nih.gov | |
Description | Data deposited in or computed by PubChem | |
InChI Key |
LBKPYHDQRHZOTL-UHFFFAOYSA-J | |
Details | Computed by InChI 1.0.5 (PubChem release 2019.06.18) | |
Source | PubChem | |
URL | https://pubchem.ncbi.nlm.nih.gov | |
Description | Data deposited in or computed by PubChem | |
Canonical SMILES |
O.F[Ce](F)(F)F | |
Details | Computed by OEChem 2.1.5 (PubChem release 2019.06.18) | |
Source | PubChem | |
URL | https://pubchem.ncbi.nlm.nih.gov | |
Description | Data deposited in or computed by PubChem | |
Molecular Formula |
CeF4H2O | |
Details | Computed by PubChem 2.1 (PubChem release 2019.06.18) | |
Source | PubChem | |
URL | https://pubchem.ncbi.nlm.nih.gov | |
Description | Data deposited in or computed by PubChem | |
Molecular Weight |
234.125 g/mol | |
Details | Computed by PubChem 2.1 (PubChem release 2021.08.13) | |
Source | PubChem | |
URL | https://pubchem.ncbi.nlm.nih.gov | |
Description | Data deposited in or computed by PubChem | |
Retrosynthesis Analysis
AI-Powered Synthesis Planning: Our tool employs the Template_relevance Pistachio, Template_relevance Bkms_metabolic, Template_relevance Pistachio_ringbreaker, Template_relevance Reaxys, Template_relevance Reaxys_biocatalysis model, leveraging a vast database of chemical reactions to predict feasible synthetic routes.
One-Step Synthesis Focus: Specifically designed for one-step synthesis, it provides concise and direct routes for your target compounds, streamlining the synthesis process.
Accurate Predictions: Utilizing the extensive PISTACHIO, BKMS_METABOLIC, PISTACHIO_RINGBREAKER, REAXYS, REAXYS_BIOCATALYSIS database, our tool offers high-accuracy predictions, reflecting the latest in chemical research and data.
Strategy Settings
Precursor scoring | Relevance Heuristic |
---|---|
Min. plausibility | 0.01 |
Model | Template_relevance |
Template Set | Pistachio/Bkms_metabolic/Pistachio_ringbreaker/Reaxys/Reaxys_biocatalysis |
Top-N result to add to graph | 6 |
Feasible Synthetic Routes
Disclaimer and Information on In-Vitro Research Products
Please be aware that all articles and product information presented on BenchChem are intended solely for informational purposes. The products available for purchase on BenchChem are specifically designed for in-vitro studies, which are conducted outside of living organisms. In-vitro studies, derived from the Latin term "in glass," involve experiments performed in controlled laboratory settings using cells or tissues. It is important to note that these products are not categorized as medicines or drugs, and they have not received approval from the FDA for the prevention, treatment, or cure of any medical condition, ailment, or disease. We must emphasize that any form of bodily introduction of these products into humans or animals is strictly prohibited by law. It is essential to adhere to these guidelines to ensure compliance with legal and ethical standards in research and experimentation.