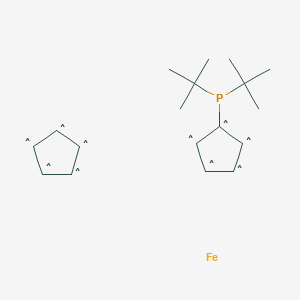
di-tert-butylphosphinoferrocene
- Click on QUICK INQUIRY to receive a quote from our team of experts.
- With the quality product at a COMPETITIVE price, you can focus more on your research.
Overview
Description
Mechanism of Action
Target of Action
Di-tert-butylphosphinoferrocene (DTBPF) is primarily used as a ligand in various metal-catalyzed reactions . Its primary targets are the metal centers of these catalysts, where it binds and influences the reactivity of the metal center .
Mode of Action
DTBPF interacts with its targets by forming a complex with the metal center of the catalyst . This interaction can influence the electronic properties of the metal center, thereby affecting its reactivity . The exact changes resulting from this interaction can vary depending on the specific reaction conditions and the other components of the catalyst system .
Biochemical Pathways
As a ligand in metal-catalyzed reactions, DTBPF can be involved in a variety of biochemical pathways. It is particularly suitable for cross-coupling reactions such as Suzuki-Miyaura Coupling, Indole Forming Reactions, Buchwald-Hartwig cross coupling, and Arylation reactions . The downstream effects of these reactions can vary widely depending on the specific substrates and reaction conditions .
Pharmacokinetics
Like other organometallic compounds, its bioavailability would likely be influenced by factors such as its solubility, stability, and reactivity .
Result of Action
The molecular and cellular effects of DTBPF’s action are primarily related to its role in facilitating metal-catalyzed reactions . By influencing the reactivity of the metal center, DTBPF can enable the formation of new chemical bonds, leading to the synthesis of a wide range of organic compounds .
Action Environment
The action, efficacy, and stability of DTBPF can be influenced by various environmental factors. For example, the presence of oxygen or moisture can potentially lead to the degradation of DTBPF or reduce its effectiveness as a ligand . Therefore, reactions involving DTBPF are often carried out under inert conditions .
Preparation Methods
The synthesis of di-tert-butylphosphinoferrocene involves several key steps. Initially, ferrocene is reacted with diarylphosphine oxide or dialkylphosphine oxide in a solvent such as 1,2-dichloroethane under controlled conditions. The reaction mixture is then subjected to hydrolysis and crystallization to obtain the final product . Industrial production methods typically involve similar steps but are optimized for higher yields and purity, often using automated systems and inert atmosphere conditions to prevent oxidation .
Chemical Reactions Analysis
Di-tert-butylphosphinoferrocene undergoes various types of chemical reactions, including:
Oxidation: The compound can be oxidized to form phosphine oxides.
Reduction: It can be reduced back to the phosphine form.
Substitution: The phosphino groups can participate in substitution reactions, particularly in the formation of metal complexes.
Common reagents used in these reactions include oxidizing agents like hydrogen peroxide for oxidation and reducing agents like lithium aluminum hydride for reduction. Major products formed from these reactions include phosphine oxides and various metal complexes .
Scientific Research Applications
Di-tert-butylphosphinoferrocene is widely used in scientific research due to its versatility as a ligand. Some of its applications include:
Biology: The compound is used in the synthesis of biologically active molecules and pharmaceuticals.
Medicine: It plays a role in the development of new drugs and therapeutic agents.
Industry: It is employed in the production of fine chemicals, polymers, and advanced materials.
Comparison with Similar Compounds
Di-tert-butylphosphinoferrocene is unique due to its bulky di-tert-butylphosphino groups, which provide steric protection and enhance the stability of its metal complexes. Similar compounds include:
1,1’-Bis(diphenylphosphino)ferrocene: This compound has phenyl groups instead of tert-butyl groups, leading to different steric and electronic properties.
1,1’-Bis(di-tert-butylphosphino)ferrocene dichloropalladium(II): This is a palladium complex of this compound, used as a catalyst in cross-coupling reactions.
These comparisons highlight the unique steric and electronic properties of this compound, making it a valuable ligand in various catalytic applications.
Properties
InChI |
InChI=1S/C13H22P.C5H5.Fe/c1-12(2,3)14(13(4,5)6)11-9-7-8-10-11;1-2-4-5-3-1;/h7-10H,1-6H3;1-5H; |
Source
|
---|---|---|
Details | Computed by InChI 1.0.5 (PubChem release 2019.06.18) | |
Source | PubChem | |
URL | https://pubchem.ncbi.nlm.nih.gov | |
Description | Data deposited in or computed by PubChem | |
InChI Key |
KMOMYOJYWXJKCJ-UHFFFAOYSA-N |
Source
|
Details | Computed by InChI 1.0.5 (PubChem release 2019.06.18) | |
Source | PubChem | |
URL | https://pubchem.ncbi.nlm.nih.gov | |
Description | Data deposited in or computed by PubChem | |
Canonical SMILES |
CC(C)(C)P([C]1[CH][CH][CH][CH]1)C(C)(C)C.[CH]1[CH][CH][CH][CH]1.[Fe] |
Source
|
Details | Computed by OEChem 2.1.5 (PubChem release 2019.06.18) | |
Source | PubChem | |
URL | https://pubchem.ncbi.nlm.nih.gov | |
Description | Data deposited in or computed by PubChem | |
Molecular Formula |
C18H27FeP |
Source
|
Details | Computed by PubChem 2.1 (PubChem release 2019.06.18) | |
Source | PubChem | |
URL | https://pubchem.ncbi.nlm.nih.gov | |
Description | Data deposited in or computed by PubChem | |
Molecular Weight |
330.2 g/mol |
Source
|
Details | Computed by PubChem 2.1 (PubChem release 2021.05.07) | |
Source | PubChem | |
URL | https://pubchem.ncbi.nlm.nih.gov | |
Description | Data deposited in or computed by PubChem | |
Disclaimer and Information on In-Vitro Research Products
Please be aware that all articles and product information presented on BenchChem are intended solely for informational purposes. The products available for purchase on BenchChem are specifically designed for in-vitro studies, which are conducted outside of living organisms. In-vitro studies, derived from the Latin term "in glass," involve experiments performed in controlled laboratory settings using cells or tissues. It is important to note that these products are not categorized as medicines or drugs, and they have not received approval from the FDA for the prevention, treatment, or cure of any medical condition, ailment, or disease. We must emphasize that any form of bodily introduction of these products into humans or animals is strictly prohibited by law. It is essential to adhere to these guidelines to ensure compliance with legal and ethical standards in research and experimentation.