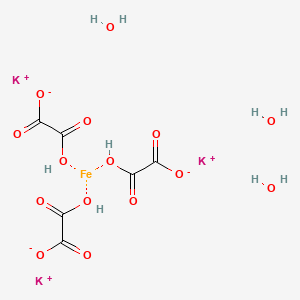
Potassium tris(oxalato)ferrate(III) trihydrate
Overview
Description
Potassium tris(oxalato)ferrate(III) trihydrate, also known as potassium ferrioxalate, is a coordination compound with the chemical formula K₃[Fe(C₂O₄)₃]·3H₂O. This compound consists of ferrioxalate anions, [Fe(C₂O₄)₃]³⁻, and potassium cations, K⁺. It is often found as emerald green crystals and is known for its stability in the dark but decomposes under light and high-energy electromagnetic radiation .
Mechanism of Action
Target of Action
Potassium trioxalatoferrate(III) trihydrate, also known as Potassium tris(oxalato)ferrate(III) trihydrate, is a complex compound containing iron(III) and potassium ions . The primary targets of this compound are the iron(III) ions, which play a crucial role in its photochemical properties .
Mode of Action
The compound is photosensitive, meaning it reacts to light of specific wavelengths . Upon exposure to light of an appropriate wavelength (less than 450 nm), the iron(III) ion in the Fe(C2O4)3 -3 complex undergoes an intramolecular redox reaction . This reaction results in the reduction of the iron(III) ion to iron(II), while one of the oxalate groups is oxidized to CO2 .
Biochemical Pathways
The biochemical pathways affected by this compound are primarily related to its photochemical properties. The intramolecular redox reaction triggered by light exposure can lead to changes in the oxidation states of the involved ions . .
Result of Action
The primary result of the compound’s action is the reduction of iron(III) to iron(II) and the oxidation of one of the oxalate groups to CO2 . This reaction can have various applications, such as in chemical actinometry, where it is used to measure light flux .
Action Environment
The action of Potassium trioxalatoferrate(III) trihydrate is influenced by environmental factors such as light and temperature . Its photosensitivity means that light exposure can trigger its redox reaction . Additionally, the compound is relatively stable under normal conditions but decomposes when heated . It is also hygroscopic and slightly soluble in water , which can affect its stability and reactivity.
Preparation Methods
Synthetic Routes and Reaction Conditions: Potassium tris(oxalato)ferrate(III) trihydrate can be synthesized through various methods. One common method involves the reaction of iron(III) sulfate, barium oxalate, and potassium oxalate in water. The reaction is as follows :
[ \text{Fe}_2(\text{SO}_4)_3 + 3 \text{BaC}_2\text{O}_4 + 3 \text{K}_2\text{C}_2\text{O}_4 \rightarrow 2 \text{K}_3[\text{Fe}(\text{C}_2\text{O}_4)_3] + 3 \text{BaSO}_4 ]
The mixture is digested for several hours on a steam bath, allowing the oxalate ions to replace the sulfate ions, forming barium sulfate, which is then filtered out. The pure this compound can be crystallized from the solution .
Industrial Production Methods: Industrial production methods for this compound typically involve similar chemical reactions but on a larger scale. The use of automated reactors and precise control of reaction conditions ensures high yield and purity of the final product.
Chemical Reactions Analysis
Types of Reactions: Potassium tris(oxalato)ferrate(III) trihydrate undergoes various chemical reactions, including:
Oxidation-Reduction Reactions: The ferrioxalate anion can undergo photoreduction, where Fe(III) is reduced to Fe(II) while one of the oxalate ligands is oxidized to carbon dioxide (CO₂) upon exposure to light.
Substitution Reactions: The oxalate ligands can be replaced by other ligands in coordination chemistry reactions.
Common Reagents and Conditions:
Oxidation-Reduction: Light (wavelength < 450 nm) is commonly used to induce photoreduction.
Substitution: Various ligands and appropriate solvents are used depending on the desired substitution reaction.
Major Products:
Scientific Research Applications
Potassium tris(oxalato)ferrate(III) trihydrate has numerous applications in scientific research:
Biology: The compound is used in studies involving iron metabolism and transport.
Medicine: Research into its potential use in drug delivery systems and as a contrast agent in imaging techniques is ongoing.
Comparison with Similar Compounds
Sodium ferrioxalate (Na₃[Fe(C₂O₄)₃]): Similar in structure but with sodium cations instead of potassium.
Iron(II) oxalate (FeC₂O₄): Contains iron in the +2 oxidation state and oxalate ligands.
Uniqueness: Potassium tris(oxalato)ferrate(III) trihydrate is unique due to its stability in the dark and its well-characterized photoreduction properties, making it a valuable compound in photochemical research .
Properties
IUPAC Name |
tripotassium;2-hydroxy-2-oxoacetate;iron;trihydrate | |
---|---|---|
Details | Computed by Lexichem TK 2.7.0 (PubChem release 2021.10.14) | |
Source | PubChem | |
URL | https://pubchem.ncbi.nlm.nih.gov | |
Description | Data deposited in or computed by PubChem | |
InChI |
InChI=1S/3C2H2O4.Fe.3K.3H2O/c3*3-1(4)2(5)6;;;;;;;/h3*(H,3,4)(H,5,6);;;;;3*1H2/q;;;;3*+1;;;/p-3 | |
Details | Computed by InChI 1.0.6 (PubChem release 2021.10.14) | |
Source | PubChem | |
URL | https://pubchem.ncbi.nlm.nih.gov | |
Description | Data deposited in or computed by PubChem | |
InChI Key |
ZXDSAQGUKFMVAX-UHFFFAOYSA-K | |
Details | Computed by InChI 1.0.6 (PubChem release 2021.10.14) | |
Source | PubChem | |
URL | https://pubchem.ncbi.nlm.nih.gov | |
Description | Data deposited in or computed by PubChem | |
Canonical SMILES |
C(=O)(C(=O)[O-])O.C(=O)(C(=O)[O-])O.C(=O)(C(=O)[O-])O.O.O.O.[K+].[K+].[K+].[Fe] | |
Details | Computed by OEChem 2.3.0 (PubChem release 2021.10.14) | |
Source | PubChem | |
URL | https://pubchem.ncbi.nlm.nih.gov | |
Description | Data deposited in or computed by PubChem | |
Molecular Formula |
C6H9FeK3O15 | |
Details | Computed by PubChem 2.2 (PubChem release 2021.10.14) | |
Source | PubChem | |
URL | https://pubchem.ncbi.nlm.nih.gov | |
Description | Data deposited in or computed by PubChem | |
Molecular Weight |
494.27 g/mol | |
Details | Computed by PubChem 2.2 (PubChem release 2021.10.14) | |
Source | PubChem | |
URL | https://pubchem.ncbi.nlm.nih.gov | |
Description | Data deposited in or computed by PubChem | |
Retrosynthesis Analysis
AI-Powered Synthesis Planning: Our tool employs the Template_relevance Pistachio, Template_relevance Bkms_metabolic, Template_relevance Pistachio_ringbreaker, Template_relevance Reaxys, Template_relevance Reaxys_biocatalysis model, leveraging a vast database of chemical reactions to predict feasible synthetic routes.
One-Step Synthesis Focus: Specifically designed for one-step synthesis, it provides concise and direct routes for your target compounds, streamlining the synthesis process.
Accurate Predictions: Utilizing the extensive PISTACHIO, BKMS_METABOLIC, PISTACHIO_RINGBREAKER, REAXYS, REAXYS_BIOCATALYSIS database, our tool offers high-accuracy predictions, reflecting the latest in chemical research and data.
Strategy Settings
Precursor scoring | Relevance Heuristic |
---|---|
Min. plausibility | 0.01 |
Model | Template_relevance |
Template Set | Pistachio/Bkms_metabolic/Pistachio_ringbreaker/Reaxys/Reaxys_biocatalysis |
Top-N result to add to graph | 6 |
Feasible Synthetic Routes
Disclaimer and Information on In-Vitro Research Products
Please be aware that all articles and product information presented on BenchChem are intended solely for informational purposes. The products available for purchase on BenchChem are specifically designed for in-vitro studies, which are conducted outside of living organisms. In-vitro studies, derived from the Latin term "in glass," involve experiments performed in controlled laboratory settings using cells or tissues. It is important to note that these products are not categorized as medicines or drugs, and they have not received approval from the FDA for the prevention, treatment, or cure of any medical condition, ailment, or disease. We must emphasize that any form of bodily introduction of these products into humans or animals is strictly prohibited by law. It is essential to adhere to these guidelines to ensure compliance with legal and ethical standards in research and experimentation.