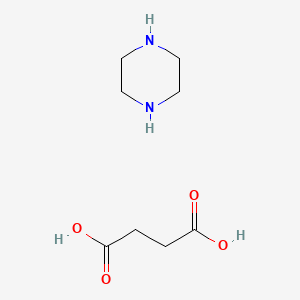
Piperazine succinate
Overview
Description
Piperazine succinate is a chemical compound that combines piperazine, a six-membered heterocyclic compound containing two nitrogen atoms at opposite positions, with succinic acid, a four-carbon dicarboxylic acid. Piperazine is known for its wide range of biological and pharmaceutical activities, making it a valuable component in various medicinal and industrial applications.
Mechanism of Action
Target of Action
Piperazine succinate primarily targets the GABA receptors in the nervous system . It binds directly and selectively to muscle membrane GABA receptors, causing hyperpolarization of nerve endings . Piperazine also targets the cytoplasmic membrane of bacteria, leading to leakage of intercellular components and cell death . Additionally, piperazine derivatives have been found to interact with sigma receptors (SRs), particularly sigma-1 (S1R) and sigma-2 (S2R) receptors .
Mode of Action
This compound acts as a GABA receptor agonist . By binding to these receptors, it causes hyperpolarization of nerve endings, resulting in flaccid paralysis of the worm . This paralysis allows the host body to easily remove or expel the invading organism. In the case of bacteria, piperazine disrupts the cytoplasmic membrane, causing leakage of intercellular components and leading to cell death .
Biochemical Pathways
The biochemical pathways affected by this compound primarily involve the GABAergic system . By acting as a GABA receptor agonist, this compound enhances the effects of GABA, a major inhibitory neurotransmitter in the nervous system. This leads to hyperpolarization of nerve endings and subsequent paralysis of the worm .
Pharmacokinetics
The pharmacokinetics of this compound involve its absorption, distribution, metabolism, and excretion (ADME) properties . Piperazine, due to its two ionizable sites, can affect the acid dissociation constant (pKa), which is crucial in absorption and distribution processes as it influences the physicochemical properties, lipophilicity, and solubility in water . More detailed studies on the ADME properties of this compound specifically are needed.
Result of Action
The primary result of this compound’s action is the paralysis and subsequent expulsion of parasitic worms from the host body . This is achieved through its agonistic action on GABA receptors, leading to hyperpolarization of nerve endings . When targeting bacteria, piperazine causes leakage of intercellular components, leading to bacterial cell death .
Action Environment
The action of this compound can be influenced by various environmental factors. For instance, the pH of the environment can affect the ionization state of piperazine, thereby influencing its absorption and distribution . Furthermore, the presence of other substances, such as other drugs or food, could potentially affect the absorption and efficacy of this compound.
Biochemical Analysis
Biochemical Properties
Piperazine succinate plays a significant role in biochemical reactions, particularly in the inhibition of acetylcholinesterase, an enzyme responsible for breaking down acetylcholine. This inhibition disrupts the transmission of nerve signals, leading to paralysis of parasitic worms . Additionally, this compound interacts with gamma-aminobutyric acid (GABA) receptors, causing hyperpolarization of nerve endings and resulting in flaccid paralysis of the worms . These interactions highlight the compound’s effectiveness as an anthelmintic agent.
Cellular Effects
This compound exerts various effects on different cell types and cellular processes. In cancer cells, piperazine derivatives have been shown to induce apoptosis by upregulating intracellular apoptotic marker proteins such as cleaved caspase-3, cytochrome c, and Bax . This compound also influences cell signaling pathways, gene expression, and cellular metabolism by interacting with GABA receptors and acetylcholinesterase . These interactions lead to changes in cell function, ultimately resulting in the death of parasitic worms or cancer cells.
Molecular Mechanism
The molecular mechanism of this compound involves its binding interactions with GABA receptors and acetylcholinesterase. By binding to GABA receptors, this compound causes hyperpolarization of nerve endings, leading to paralysis of the worms . Additionally, the inhibition of acetylcholinesterase disrupts the breakdown of acetylcholine, further contributing to the paralysis and eventual death of the parasites . These molecular interactions underscore the compound’s effectiveness as an anthelmintic agent.
Temporal Effects in Laboratory Settings
In laboratory settings, the effects of this compound have been observed to change over time. The compound’s stability and degradation are crucial factors in its long-term effectiveness. Studies have shown that this compound remains stable under various conditions, maintaining its anthelmintic properties over extended periods . Prolonged exposure to the compound may lead to degradation, potentially reducing its efficacy. Long-term effects on cellular function have been observed in both in vitro and in vivo studies, highlighting the importance of monitoring the compound’s stability and degradation over time .
Dosage Effects in Animal Models
The effects of this compound vary with different dosages in animal models. At lower doses, the compound effectively paralyzes and eliminates parasitic worms without causing significant adverse effects . At higher doses, this compound can cause neurotoxic symptoms such as salivation, vomiting, diarrhea, trembling, and convulsions . These threshold effects highlight the importance of determining the appropriate dosage to maximize efficacy while minimizing toxicity.
Metabolic Pathways
This compound is involved in various metabolic pathways, including the inhibition of succinic acid metabolism in ascarids . This inhibition disrupts the energy management of the worms, contributing to their paralysis and death. Additionally, this compound interacts with enzymes and cofactors involved in the metabolism of succinic acid, further highlighting its role in disrupting parasitic energy metabolism .
Transport and Distribution
The transport and distribution of this compound within cells and tissues involve interactions with transporters and binding proteins. Piperazine-derived lipid nanoparticles have been shown to deliver mRNA to immune cells in vivo, demonstrating the compound’s ability to interact with cellular transport mechanisms . These interactions facilitate the localization and accumulation of this compound within specific tissues, enhancing its therapeutic efficacy.
Subcellular Localization
This compound’s subcellular localization is influenced by targeting signals and post-translational modifications. The compound has been observed to localize within various cellular compartments, including the cytoplasm and mitochondria . These localizations are crucial for its activity and function, as they enable this compound to interact with specific biomolecules and exert its therapeutic effects.
Preparation Methods
Synthetic Routes and Reaction Conditions: Piperazine succinate can be synthesized through the reaction of piperazine with succinic acid. The process typically involves the following steps:
Reaction of Piperazine with Succinic Acid: Piperazine is reacted with succinic acid in a suitable solvent, such as water or ethanol, under reflux conditions. The reaction is usually carried out at elevated temperatures to ensure complete dissolution and reaction of the reactants.
Isolation and Purification: The resulting this compound is isolated by filtration or crystallization. The product is then purified by recrystallization from an appropriate solvent to obtain a high-purity compound.
Industrial Production Methods: In industrial settings, the production of this compound may involve continuous flow processes to enhance efficiency and yield. The use of automated systems and optimized reaction conditions ensures consistent quality and scalability of the compound.
Chemical Reactions Analysis
Types of Reactions: Piperazine succinate undergoes various chemical reactions, including:
Oxidation: this compound can be oxidized using oxidizing agents such as hydrogen peroxide or potassium permanganate to form corresponding oxidized products.
Reduction: Reduction reactions involving this compound can be carried out using reducing agents like sodium borohydride or lithium aluminum hydride.
Substitution: this compound can undergo nucleophilic substitution reactions, where the nitrogen atoms in the piperazine ring act as nucleophiles, reacting with electrophiles to form substituted derivatives.
Common Reagents and Conditions:
Oxidation: Hydrogen peroxide, potassium permanganate, and other oxidizing agents under acidic or basic conditions.
Reduction: Sodium borohydride, lithium aluminum hydride, and other reducing agents under anhydrous conditions.
Substitution: Electrophiles such as alkyl halides, acyl chlorides, and sulfonyl chlorides in the presence of a base or catalyst.
Major Products Formed:
Oxidation: Oxidized derivatives of this compound.
Reduction: Reduced derivatives of this compound.
Substitution: Substituted this compound derivatives with various functional groups.
Scientific Research Applications
Piperazine succinate has a wide range of applications in scientific research, including:
Chemistry: Used as a building block in the synthesis of various heterocyclic compounds and pharmaceutical intermediates.
Biology: Employed in the study of enzyme inhibition and receptor binding due to its structural similarity to biologically active molecules.
Medicine: Utilized in the development of anthelmintic drugs for the treatment of parasitic infections such as roundworm and pinworm.
Industry: Applied in the production of polymers, resins, and other industrial chemicals due to its reactivity and stability.
Comparison with Similar Compounds
Piperazine succinate can be compared with other similar compounds, such as:
Piperazine citrate: Another piperazine derivative used as an anthelmintic agent. Both compounds share similar mechanisms of action but differ in their salt forms and solubility properties.
Piperazine hydrate: A hydrated form of piperazine used in various pharmaceutical applications. It has similar biological activities but different physical properties compared to this compound.
Morpholine: A six-membered heterocyclic compound with one nitrogen and one oxygen atom. While morpholine shares some structural similarities with piperazine, it has different chemical reactivity and applications.
This compound is unique due to its combination of piperazine and succinic acid, which imparts specific chemical and biological properties that are valuable in various applications.
Properties
IUPAC Name |
butanedioic acid;piperazine | |
---|---|---|
Details | Computed by Lexichem TK 2.7.0 (PubChem release 2021.05.07) | |
Source | PubChem | |
URL | https://pubchem.ncbi.nlm.nih.gov | |
Description | Data deposited in or computed by PubChem | |
InChI |
InChI=1S/C4H10N2.C4H6O4/c1-2-6-4-3-5-1;5-3(6)1-2-4(7)8/h5-6H,1-4H2;1-2H2,(H,5,6)(H,7,8) | |
Details | Computed by InChI 1.0.6 (PubChem release 2021.05.07) | |
Source | PubChem | |
URL | https://pubchem.ncbi.nlm.nih.gov | |
Description | Data deposited in or computed by PubChem | |
InChI Key |
ROEOHFSDZDGESV-UHFFFAOYSA-N | |
Details | Computed by InChI 1.0.6 (PubChem release 2021.05.07) | |
Source | PubChem | |
URL | https://pubchem.ncbi.nlm.nih.gov | |
Description | Data deposited in or computed by PubChem | |
Canonical SMILES |
C1CNCCN1.C(CC(=O)O)C(=O)O | |
Details | Computed by OEChem 2.3.0 (PubChem release 2021.05.07) | |
Source | PubChem | |
URL | https://pubchem.ncbi.nlm.nih.gov | |
Description | Data deposited in or computed by PubChem | |
Molecular Formula |
C8H16N2O4 | |
Details | Computed by PubChem 2.1 (PubChem release 2021.05.07) | |
Source | PubChem | |
URL | https://pubchem.ncbi.nlm.nih.gov | |
Description | Data deposited in or computed by PubChem | |
Molecular Weight |
204.22 g/mol | |
Details | Computed by PubChem 2.1 (PubChem release 2021.05.07) | |
Source | PubChem | |
URL | https://pubchem.ncbi.nlm.nih.gov | |
Description | Data deposited in or computed by PubChem | |
CAS No. |
14396-13-5 | |
Record name | Butanedioic acid, compd. with piperazine (1:1) | |
Source | CAS Common Chemistry | |
URL | https://commonchemistry.cas.org/detail?cas_rn=14396-13-5 | |
Description | CAS Common Chemistry is an open community resource for accessing chemical information. Nearly 500,000 chemical substances from CAS REGISTRY cover areas of community interest, including common and frequently regulated chemicals, and those relevant to high school and undergraduate chemistry classes. This chemical information, curated by our expert scientists, is provided in alignment with our mission as a division of the American Chemical Society. | |
Explanation | The data from CAS Common Chemistry is provided under a CC-BY-NC 4.0 license, unless otherwise stated. | |
Retrosynthesis Analysis
AI-Powered Synthesis Planning: Our tool employs the Template_relevance Pistachio, Template_relevance Bkms_metabolic, Template_relevance Pistachio_ringbreaker, Template_relevance Reaxys, Template_relevance Reaxys_biocatalysis model, leveraging a vast database of chemical reactions to predict feasible synthetic routes.
One-Step Synthesis Focus: Specifically designed for one-step synthesis, it provides concise and direct routes for your target compounds, streamlining the synthesis process.
Accurate Predictions: Utilizing the extensive PISTACHIO, BKMS_METABOLIC, PISTACHIO_RINGBREAKER, REAXYS, REAXYS_BIOCATALYSIS database, our tool offers high-accuracy predictions, reflecting the latest in chemical research and data.
Strategy Settings
Precursor scoring | Relevance Heuristic |
---|---|
Min. plausibility | 0.01 |
Model | Template_relevance |
Template Set | Pistachio/Bkms_metabolic/Pistachio_ringbreaker/Reaxys/Reaxys_biocatalysis |
Top-N result to add to graph | 6 |
Feasible Synthetic Routes
Disclaimer and Information on In-Vitro Research Products
Please be aware that all articles and product information presented on BenchChem are intended solely for informational purposes. The products available for purchase on BenchChem are specifically designed for in-vitro studies, which are conducted outside of living organisms. In-vitro studies, derived from the Latin term "in glass," involve experiments performed in controlled laboratory settings using cells or tissues. It is important to note that these products are not categorized as medicines or drugs, and they have not received approval from the FDA for the prevention, treatment, or cure of any medical condition, ailment, or disease. We must emphasize that any form of bodily introduction of these products into humans or animals is strictly prohibited by law. It is essential to adhere to these guidelines to ensure compliance with legal and ethical standards in research and experimentation.