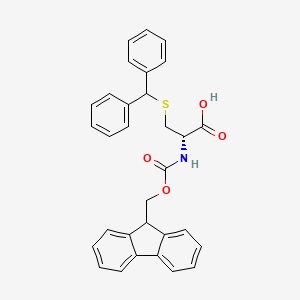
Fmoc-D-Cys(Dpm)-OH
- Click on QUICK INQUIRY to receive a quote from our team of experts.
- With the quality product at a COMPETITIVE price, you can focus more on your research.
Overview
Description
Fmoc-D-Cys(Dpm)-OH, also known as Fmoc-protected cysteine, is a synthetic amino acid used in peptide synthesis. It is a derivative of cysteine, an essential amino acid found in proteins, and is used to produce peptides with improved stability and solubility. This compound is a valuable tool in many scientific research applications, including peptide synthesis, protein engineering, and drug discovery.
Scientific Research Applications
Fmoc-D-Cys(Dpm)-OH is used in a variety of scientific research applications. It is used in peptide synthesis to produce peptides with improved stability and solubility. It is also used in protein engineering to create proteins with modified structures and functions. In addition, this compound is used in drug discovery, as it can be used to modify the structure of drug molecules to improve their properties.
Mechanism of Action
The mechanism of action of Fmoc-D-Cys(Dpm)-OH is based on its ability to form covalent bonds with other molecules. The Fmoc group is a protecting group that is used to protect the cysteine residue from unwanted reactions during peptide synthesis. When the Fmoc group is removed, the cysteine residue is deprotected and can react with other molecules, forming covalent bonds.
Biochemical and Physiological Effects
The biochemical and physiological effects of this compound are not well understood, as it is primarily used in laboratory experiments. However, it is known that this compound can be used to modify the structure of proteins, and this can have an effect on the protein’s function. In addition, this compound can be used to modify the structure of drug molecules, which can affect their pharmacological properties.
Advantages and Limitations for Lab Experiments
The main advantage of using Fmoc-D-Cys(Dpm)-OH in laboratory experiments is its ability to form covalent bonds with other molecules. This allows researchers to modify the structure of proteins and drug molecules, which can have a significant impact on their properties. However, there are some limitations to using this compound in laboratory experiments. For example, it can be difficult to remove the Fmoc group from the cysteine residue, and this can limit the accuracy of the experiment.
Future Directions
There are a number of potential future directions for research related to Fmoc-D-Cys(Dpm)-OH. These include exploring new methods for synthesizing Fmoc-D-Cys(Dpm)-OHed cysteine, developing new methods for modifying proteins and drug molecules using this compound, and investigating the biochemical and physiological effects of this compound. In addition, researchers could investigate the potential applications of this compound in biotechnology and medical research.
Synthesis Methods
Fmoc-D-Cys(Dpm)-OH is synthesized using a solid-phase peptide synthesis (SPPS) method. In this method, a peptide is synthesized on a solid support, such as a resin, and the Fmoc-D-Cys(Dpm)-OHed cysteine is incorporated into the peptide chain. The Fmoc group is then removed from the cysteine residue, allowing the cysteine to be deprotected and reacted with the other amino acids in the peptide chain.
properties
IUPAC Name |
(2S)-3-benzhydrylsulfanyl-2-(9H-fluoren-9-ylmethoxycarbonylamino)propanoic acid |
Source
|
---|---|---|
Details | Computed by Lexichem TK 2.7.0 (PubChem release 2021.05.07) | |
Source | PubChem | |
URL | https://pubchem.ncbi.nlm.nih.gov | |
Description | Data deposited in or computed by PubChem | |
InChI |
InChI=1S/C31H27NO4S/c33-30(34)28(20-37-29(21-11-3-1-4-12-21)22-13-5-2-6-14-22)32-31(35)36-19-27-25-17-9-7-15-23(25)24-16-8-10-18-26(24)27/h1-18,27-29H,19-20H2,(H,32,35)(H,33,34)/t28-/m1/s1 |
Source
|
Details | Computed by InChI 1.0.6 (PubChem release 2021.05.07) | |
Source | PubChem | |
URL | https://pubchem.ncbi.nlm.nih.gov | |
Description | Data deposited in or computed by PubChem | |
InChI Key |
IVPLDYIPIHKRET-MUUNZHRXSA-N |
Source
|
Details | Computed by InChI 1.0.6 (PubChem release 2021.05.07) | |
Source | PubChem | |
URL | https://pubchem.ncbi.nlm.nih.gov | |
Description | Data deposited in or computed by PubChem | |
Canonical SMILES |
C1=CC=C(C=C1)C(C2=CC=CC=C2)SCC(C(=O)O)NC(=O)OCC3C4=CC=CC=C4C5=CC=CC=C35 |
Source
|
Details | Computed by OEChem 2.3.0 (PubChem release 2021.05.07) | |
Source | PubChem | |
URL | https://pubchem.ncbi.nlm.nih.gov | |
Description | Data deposited in or computed by PubChem | |
Isomeric SMILES |
C1=CC=C(C=C1)C(C2=CC=CC=C2)SC[C@H](C(=O)O)NC(=O)OCC3C4=CC=CC=C4C5=CC=CC=C35 |
Source
|
Details | Computed by OEChem 2.3.0 (PubChem release 2021.05.07) | |
Source | PubChem | |
URL | https://pubchem.ncbi.nlm.nih.gov | |
Description | Data deposited in or computed by PubChem | |
Molecular Formula |
C31H27NO4S |
Source
|
Details | Computed by PubChem 2.1 (PubChem release 2021.05.07) | |
Source | PubChem | |
URL | https://pubchem.ncbi.nlm.nih.gov | |
Description | Data deposited in or computed by PubChem | |
Molecular Weight |
509.6 g/mol |
Source
|
Details | Computed by PubChem 2.1 (PubChem release 2021.05.07) | |
Source | PubChem | |
URL | https://pubchem.ncbi.nlm.nih.gov | |
Description | Data deposited in or computed by PubChem | |
Disclaimer and Information on In-Vitro Research Products
Please be aware that all articles and product information presented on BenchChem are intended solely for informational purposes. The products available for purchase on BenchChem are specifically designed for in-vitro studies, which are conducted outside of living organisms. In-vitro studies, derived from the Latin term "in glass," involve experiments performed in controlled laboratory settings using cells or tissues. It is important to note that these products are not categorized as medicines or drugs, and they have not received approval from the FDA for the prevention, treatment, or cure of any medical condition, ailment, or disease. We must emphasize that any form of bodily introduction of these products into humans or animals is strictly prohibited by law. It is essential to adhere to these guidelines to ensure compliance with legal and ethical standards in research and experimentation.