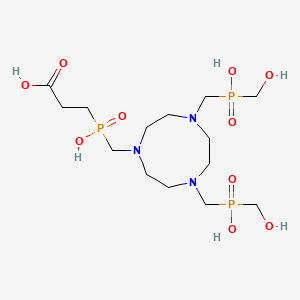
NOPO
Overview
Description
NOPO is referenced in multiple scientific contexts, each representing distinct chemical or biological entities. This article focuses on two primary classifications where this compound is compared to analogous compounds:
Preparation Methods
The synthesis of NOPO involves several steps, typically starting with the nitration of propanol. The process can be summarized as follows:
Nitration of Propanol: Propanol is treated with a nitrating agent, such as nitric acid, to introduce a nitro group, forming 3-nitropropanol.
Oxidation: The 3-nitropropanol is then oxidized to form 3-nitrooxypropanol.
Industrial production methods for this compound are designed to be efficient and scalable, ensuring that the compound can be produced in large quantities to meet demand. These methods often involve continuous flow processes and advanced purification techniques to achieve high purity levels .
Chemical Reactions Analysis
NOPO undergoes various chemical reactions, including:
Oxidation: this compound can be further oxidized to form other nitro compounds. Common oxidizing agents include hydrogen peroxide and peracids.
Reduction: this compound can be reduced to form 3-aminopropanol using reducing agents such as lithium aluminum hydride.
Substitution: this compound can undergo nucleophilic substitution reactions, where the nitro group is replaced by other functional groups.
The major products formed from these reactions depend on the specific conditions and reagents used. For example, reduction of this compound typically yields 3-aminopropanol, while substitution reactions can produce a variety of derivatives depending on the nucleophile employed .
Scientific Research Applications
NOPO in Radiopharmaceuticals
Chelation Properties
This compound has been prominently utilized as a chelator for Gallium-68 in radiopharmaceuticals. Its favorable labeling properties make it highly recommendable for the reliable routine production of -radiopharmaceuticals, which are crucial for positron emission tomography (PET) imaging. The efficiency of this compound in this application stems from its ability to form stable complexes with Gallium-68, facilitating effective radiolabeling processes .
Case Study: Gallium-68 Peptides
A study highlighted the benefits of this compound as a chelator in the synthesis of -labeled peptides. The research demonstrated that this compound conjugates significantly enhance the yield and stability of radiolabeled compounds, making them suitable for clinical applications in oncology .
This compound and the Nociceptin Opioid Receptor System
Therapeutic Potential
The nociceptin opioid receptor (NOP) system, which includes the endogenous peptide nociceptin/orphanin FQ (N/OFQ), has been explored for its therapeutic potential in various conditions such as pain management, anxiety disorders, and substance abuse treatment. Recent findings suggest that NOP receptor agonists may provide benefits in treating chronic pain and reducing opioid dependence .
Clinical Trials and Research Findings
- Cebranopadol : This dual-targeted NOP agonist/MOP agonist is currently in Phase III clinical trials for pain treatment. Its development signifies a promising direction for NOP-targeted therapies .
- LY2940094 : An NOP antagonist under investigation for major depressive disorder and alcohol dependence is in Phase II clinical trials, showcasing the broader implications of targeting the NOP system .
Applications in Nanotechnology
SWCNT Production
This compound also plays a role in nanotechnology, particularly in the production of single-walled carbon nanotubes (SWCNTs). Recent funding initiatives have highlighted over 100 applications being developed globally, ranging from cancer treatment to desalination processes. The scalability of SWCNT production using this compound-based methods is expected to enhance the material's availability for diverse industrial applications .
Data Summary Table
Application Area | Compound Type | Clinical Status | Key Findings |
---|---|---|---|
Radiopharmaceuticals | Gallium-68 Peptides | Approved for use | High yield and stability in PET imaging |
Pain Management | Cebranopadol | Phase III Trials | Dual-targeted approach shows promise |
Mental Health | LY2940094 | Phase II Trials | Potential treatment for depression and addiction |
Nanotechnology | SWCNTs | Research Phase | Over 100 applications identified |
Mechanism of Action
The primary mechanism by which NOPO exerts its effects is through the inhibition of the enzyme methyl-coenzyme M reductase, which is involved in the final step of methane production in methanogenic archaea. By binding to the active site of this enzyme, this compound effectively reduces methane production in ruminants . This mechanism is particularly valuable in efforts to reduce greenhouse gas emissions from livestock .
Comparison with Similar Compounds
NOPO as a Supramolecular Ligand
This compound (referred to as G("this compound") in ) is a phosphorus-containing ligand designed for the selective extraction of lanthanide and actinide ions. It belongs to a broader class of compounds featuring diverse -R groups (e.g., phenyl, alkyl, amides) that modulate extraction efficiency .
This compound as a Botanical Pesticide
This compound is also an emulsifiable concentrate (EC) formulation combining neem oil (NO) and pungam oil (PO). It demonstrates significant efficacy against the rice leaffolder (Cnaphalocrocis medinalis), acting as an antifeedant and growth inhibitor .
Comparative Analysis of this compound with Similar Compounds
This compound as a Supramolecular Ligand
Comparison Basis : Structural modifications (R-groups) and extraction performance for f-block elements.
Key Findings:
- G("this compound") and H("NOPOPO") are structurally related ligands with tailored R-groups for enhanced selectivity.
- Ligands with phenyl rings (e.g., compound 64–67 in ) show moderate efficiency, while trifluoromethyl-substituted benzenes (72 ) improve stability in acidic conditions.
- G("this compound") balances extraction capacity and solubility, making it versatile for industrial separation processes .
Table 1: Comparison of Supramolecular Ligands
This compound as a Botanical Pesticide
Comparison Basis: Efficacy metrics (larval mortality, pupation inhibition) against neem oil (NO) and other formulations.
Key Findings:
- This compound 60 EC (C) 3% outperforms standalone neem oil (NO 60 EC) by 20–30% in larval mortality and pupal weight reduction.
- Synergistic effects between neem and pungam oils in this compound disrupt insect lipid metabolism and oviposition behavior .
Table 2: Efficacy of this compound vs. Similar Pesticides
Critical Observations
- Structural Flexibility in Ligands: Modifying R-groups in this compound derivatives optimizes metal-ion selectivity, though trade-offs between solubility and binding strength exist .
- Synergy in Pesticides: this compound’s dual-oil formulation highlights the importance of combinatorial botanical agents for sustainable pest control .
Biological Activity
NOPO, the Drosophila ortholog of the TRAF-interacting protein (TRIP), plays a significant role in various biological processes, particularly in cell death regulation and genomic stability. This article explores the biological activity of this compound, focusing on its mechanisms of action, case studies, and research findings.
This compound functions primarily as an E3 ubiquitin ligase , which is crucial for the ubiquitylation process that regulates protein degradation and cellular signaling. Research has shown that this compound modulates Egr-induced cell death via a caspase-dependent but JNK-independent pathway. In Drosophila models, the expression of this compound was linked to the regulation of pro-apoptotic genes, influencing cell survival and death pathways.
Key Findings:
- Caspase Activation : this compound enhances caspase activation during Egr-triggered apoptosis, as demonstrated in several genetic screens where mutations affecting this compound altered cell death responses .
- Genomic Stability : this compound is involved in maintaining genomic integrity during early embryogenesis. It promotes the ubiquitylation of DNA polymerase η (Polη), facilitating translesion synthesis, which is critical for bypassing DNA damage during replication .
Data Table: Summary of this compound's Biological Functions
Case Study 1: Egr-Induced Cell Death
A study conducted by Merkle et al. (2009) utilized genetic screens to identify modifiers of Egr-induced cell death in Drosophila. The study revealed that overexpression of this compound could rescue defective eye phenotypes caused by Egr activation, confirming its role in apoptosis regulation through caspase pathways.
Case Study 2: Genomic Maintenance
In another investigation, researchers examined embryos from This compound mutant females, which exhibited mitotic arrest due to incomplete DNA replication. This study highlighted the importance of this compound in ensuring proper S-phase duration before mitosis, thereby preventing genomic instability .
Research Findings
- Ubiquitin Ligase Activity : this compound's role as an E3 ubiquitin ligase was confirmed through co-immunoprecipitation studies that demonstrated its interaction with Polη. This interaction is vital for the regulation of DNA repair mechanisms .
- Impact on Development : The absence of functional this compound leads to severe developmental defects in Drosophila embryos, including failure to progress past early developmental stages due to mitotic errors triggered by unreplicated DNA .
- Potential Therapeutic Insights : Understanding this compound's mechanisms may provide insights into therapeutic strategies for diseases characterized by dysregulated apoptosis or genomic instability, such as cancer.
Q & A
Q. NOPO as a Gallium(III) Chelator in Radiopharmaceutical Development
Basic Questions
Q. What structural features of this compound contribute to its high Ga(III) complexation efficiency, and how can researchers validate this experimentally?
this compound's tris-phosphinic acid structure provides strong coordination sites for Ga(III), achieving a stability constant (log KGaL) of 25.0 . Researchers can validate complexation efficiency via potentiometric titration to determine stepwise protonation constants (e.g., log Ka values: 11.96, 5.22, 3.77, 1.54) and assess labeling efficiency using radiometric assays. For example, at pH 3–4, >95% <sup>68</sup>Ga incorporation occurs within 5 minutes at room temperature, verified via radio-HPLC .
Q. How does this compound compare to traditional chelators (e.g., DOTA, NOTA) in terms of pharmacokinetics for in vivo applications?
this compound conjugates exhibit superior hydrophilicity (log D = -4.6 vs. -3.2 for DOTA analogs), promoting rapid renal clearance and reducing nonspecific tissue uptake. Methodologically, researchers quantify biodistribution in xenograft models (e.g., AR42J tumors) using γ-counting and PET imaging. For instance, <sup>68</sup>Ga-NOPO-NOC shows tumor uptake of 13.9 ± 5% ID/g at 120 minutes, with blockable specificity confirmed via competitive assays .
Advanced Questions 3. Q. What strategies mitigate phosphilactone formation during this compound bioconjugation, and how do these affect radiolabeling protocols? Phosphilactone formation during amide bond synthesis (e.g., HATU coupling) is minimized by pre-complexing this compound with Zn(II), which preserves the carboxylate for conjugation without hindering subsequent <sup>68</sup>Ga labeling. Researchers validate this via LC-MS to confirm conjugate integrity and monitor phosphilactone cleavage under acidic conditions (e.g., trifluoroacetic acid) .
Q. How can researchers optimize this compound-based probes for dual-modality imaging (e.g., PET/MRI) while maintaining high specific activity?
Co-labeling this compound with Ga-68 and MRI-active metals (e.g., Mn(II)) requires evaluating competitive binding via ICP-MS. Preclinical studies using αvβ3-targeted this compound-c(RGDfK) demonstrate specific activities up to 6 TBq/μmol, achieved by minimizing precursor amounts (0.5 nmol) during automated synthesis .
Q. II. This compound as a Non-degenerate Optical Parametric Oscillator (Quantum Optics)
Basic Questions 5. Q. What experimental parameters are critical for generating two-mode entanglement using a non-degenerate optical parametric oscillator (this compound)? Key parameters include pump power thresholds, cavity detuning (Δ), and seed injection ratios (ISR). For example, entanglement persists even at ISR = 0.7 when seeds are injected through the output coupler, verified via quantum correlation spectra and homodyne detection .
Q. How do researchers quantify entanglement quality in this compound systems, and what metrics are used?
Entanglement is quantified using the logarithmic negativity (EN) or two-mode squeezing levels. Theoretical models solve Langevin equations under different detuning conditions, while experimental setups measure quadrature variances using balanced detectors .
Advanced Questions 7. Q. How do detunings in this compound systems impact entanglement robustness in gravitational wave detection applications? Detunings induce phase-sensitive amplification, altering squeezing spectra. Researchers model detuning effects using coupled-mode equations and validate via optomechanical setups. For instance, detuned NOPOs enhance sensitivity beyond standard quantum limits by leveraging optical spring effects .
Q. What methodologies resolve contradictions between theoretical predictions and experimental entanglement data in detuned this compound systems?
Discrepancies arise from unaccounted thermal fluctuations or nonlinear losses. Researchers employ stochastic simulations (Fokker-Planck equations) and cross-validate with experimental spectra under controlled temperature and vacuum conditions .
Q. III. This compound in Developmental Biology (Drosophila Studies)
Basic Questions 9. Q. What experimental approaches confirm this compound's role in caspase-mediated cell death in Drosophila? RNAi knockdown of This compound in Drosophila eye or wing discs (e.g., GMR>this compound or sd>this compound systems) suppresses Egr-induced cell death phenotypes. Caspase activation is validated via TUNEL assays and immunostaining for cleaved Caspase-3 .
Advanced Questions 10. Q. How do researchers investigate this compound's interaction with DNA checkpoint kinases (e.g., CHK2) during mitotic regulation? Genetic suppression experiments show that CHK2 mutation rescues mitotic arrest in This compound mutants. Yeast two-hybrid assays and co-immunoprecipitation identify physical interactions between this compound and Bendless (E2 ubiquitin-conjugating enzyme), linking this compound to DNA replication checkpoints .
Q. Methodological Best Practices
- Data Contradictions : When stability constants (e.g., log KGaL) conflict across studies, validate via independent methods like isothermal titration calorimetry (ITC) or X-ray crystallography .
- Experimental Design : For quantum optics, use phase-locked seed injection to stabilize this compound output and reduce phase noise .
- Biological Replicates : In Drosophila studies, analyze ≥3 independent RNAi lines to control for off-target effects .
Properties
IUPAC Name |
3-[[4,7-bis[[hydroxy(hydroxymethyl)phosphoryl]methyl]-1,4,7-triazonan-1-yl]methyl-hydroxyphosphoryl]propanoic acid | |
---|---|---|
Details | Computed by Lexichem TK 2.7.0 (PubChem release 2021.05.07) | |
Source | PubChem | |
URL | https://pubchem.ncbi.nlm.nih.gov | |
Description | Data deposited in or computed by PubChem | |
InChI |
InChI=1S/C14H32N3O10P3/c18-12-29(24,25)10-16-4-2-15(9-28(22,23)8-1-14(20)21)3-5-17(7-6-16)11-30(26,27)13-19/h18-19H,1-13H2,(H,20,21)(H,22,23)(H,24,25)(H,26,27) | |
Details | Computed by InChI 1.0.6 (PubChem release 2021.05.07) | |
Source | PubChem | |
URL | https://pubchem.ncbi.nlm.nih.gov | |
Description | Data deposited in or computed by PubChem | |
InChI Key |
ZNYVGVMHKCUCAT-UHFFFAOYSA-N | |
Details | Computed by InChI 1.0.6 (PubChem release 2021.05.07) | |
Source | PubChem | |
URL | https://pubchem.ncbi.nlm.nih.gov | |
Description | Data deposited in or computed by PubChem | |
Canonical SMILES |
C1CN(CCN(CCN1CP(=O)(CCC(=O)O)O)CP(=O)(CO)O)CP(=O)(CO)O | |
Details | Computed by OEChem 2.3.0 (PubChem release 2021.05.07) | |
Source | PubChem | |
URL | https://pubchem.ncbi.nlm.nih.gov | |
Description | Data deposited in or computed by PubChem | |
Molecular Formula |
C14H32N3O10P3 | |
Details | Computed by PubChem 2.1 (PubChem release 2021.05.07) | |
Source | PubChem | |
URL | https://pubchem.ncbi.nlm.nih.gov | |
Description | Data deposited in or computed by PubChem | |
Molecular Weight |
495.34 g/mol | |
Details | Computed by PubChem 2.1 (PubChem release 2021.05.07) | |
Source | PubChem | |
URL | https://pubchem.ncbi.nlm.nih.gov | |
Description | Data deposited in or computed by PubChem | |
Retrosynthesis Analysis
AI-Powered Synthesis Planning: Our tool employs the Template_relevance Pistachio, Template_relevance Bkms_metabolic, Template_relevance Pistachio_ringbreaker, Template_relevance Reaxys, Template_relevance Reaxys_biocatalysis model, leveraging a vast database of chemical reactions to predict feasible synthetic routes.
One-Step Synthesis Focus: Specifically designed for one-step synthesis, it provides concise and direct routes for your target compounds, streamlining the synthesis process.
Accurate Predictions: Utilizing the extensive PISTACHIO, BKMS_METABOLIC, PISTACHIO_RINGBREAKER, REAXYS, REAXYS_BIOCATALYSIS database, our tool offers high-accuracy predictions, reflecting the latest in chemical research and data.
Strategy Settings
Precursor scoring | Relevance Heuristic |
---|---|
Min. plausibility | 0.01 |
Model | Template_relevance |
Template Set | Pistachio/Bkms_metabolic/Pistachio_ringbreaker/Reaxys/Reaxys_biocatalysis |
Top-N result to add to graph | 6 |
Feasible Synthetic Routes
Disclaimer and Information on In-Vitro Research Products
Please be aware that all articles and product information presented on BenchChem are intended solely for informational purposes. The products available for purchase on BenchChem are specifically designed for in-vitro studies, which are conducted outside of living organisms. In-vitro studies, derived from the Latin term "in glass," involve experiments performed in controlled laboratory settings using cells or tissues. It is important to note that these products are not categorized as medicines or drugs, and they have not received approval from the FDA for the prevention, treatment, or cure of any medical condition, ailment, or disease. We must emphasize that any form of bodily introduction of these products into humans or animals is strictly prohibited by law. It is essential to adhere to these guidelines to ensure compliance with legal and ethical standards in research and experimentation.