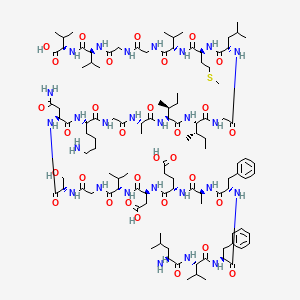
Amyloid b-Protein (17-40) Ammonium
Overview
Description
Amyloid b-Protein (17-40) Ammonium is a peptide fragment derived from the amyloid precursor protein. This compound is significant in the study of neurodegenerative diseases, particularly Alzheimer’s disease, due to its role in the formation of amyloid plaques. These plaques are a hallmark of Alzheimer’s disease and are associated with neuronal damage and cognitive decline.
Mechanism of Action
Target of Action
Amyloid β-Protein (17-40) Ammonium, also known as Aβ (17-40), is a fragment of the larger Amyloid β-Protein (Aβ). The primary targets of Aβ (17-40) are neurons in the brain . Aβ peptides, including Aβ (17-40), are generated from the amyloid precursor protein (APP) through sequential cleavage of β- and γ-secretases . The role of these peptides is critical in the progression of Alzheimer’s Disease (AD), where they accumulate and aggregate, leading to neuronal damage .
Mode of Action
Aβ (17-40) interacts with its neuronal targets, leading to several changes. At physiological levels, Aβ enhances synaptic plasticity and learning and memory in animals by promoting long-term potentiation (LTP) in the hippocampus. This action involves increasing the release of the neurotransmitter acetylcholine and activation of nicotinic acetylcholine receptors . At high (pathological) doses, aβ can inhibit memory .
Biochemical Pathways
The accumulation of Aβ peptides, including Aβ (17-40), can trigger various biochemical pathways. For instance, Aβ (17-40) and Aβ (17-42) have been found to employ distinct molecular pathways for cell entry and intracellular transit at the blood-brain barrier (BBB) . Aβ (17-40) has also been associated with the formation of distinct transient structures that gradually rearrange into protofibrils .
Pharmacokinetics
Studies on similar aβ peptides suggest that they can cross the bbb, which is a critical portal regulating the bidirectional transport of aβ proteins between blood and brain . The BBB-penetrating bispecific antibody displayed higher interstitial fluid (ISF) concentrations than the monospecific antibody .
Result of Action
The result of Aβ (17-40) action is complex and depends on its concentration. At physiological levels, it enhances synaptic plasticity and learning and memory in animals . At high (pathological) doses, aβ can inhibit memory . Moreover, the accumulation of Aβ peptides, including Aβ (17-40), can lead to neuronal damage, a characteristic feature of AD .
Action Environment
The action, efficacy, and stability of Aβ (17-40) are influenced by various environmental factors. For instance, the conformation of Aβ species is stabilized by intra- and inter-molecular hydrogen bonds and by electrostatic and hydrophobic interactions, all depending on the chemical and physical environment (e.g., solvent, ions, pH) and interactions with other molecules, such as lipids and proteins .
Biochemical Analysis
Biochemical Properties
Amyloid b-Protein (17-40) Ammonium interacts with various biomolecules, including enzymes and proteins. It is known to bind to lipoproteins and apolipoproteins E and J in the cerebrospinal fluid (CSF) and to high-density lipoprotein (HDL) particles in plasma . This binding inhibits metal-catalyzed oxidation of lipoproteins .
Cellular Effects
This compound has significant effects on various types of cells and cellular processes. It is known to activate mononuclear phagocytes in the brain and elicit inflammatory responses . It also has antimicrobial activity, can block leaks in the blood-brain barrier, enhance recovery from posttraumatic brain injury, and possibly suppress cancer through inhibition of oncogenic viruses .
Molecular Mechanism
The molecular mechanism of action of this compound involves binding interactions with biomolecules and changes in gene expression. It has been found that the extension domain of secreted Amyloid b-Protein can bind to the intrinsically disordered sushi 1 domain of the γ-aminobutyric acid type B receptor subunit 1a (GABA B R1a) and modulate its synaptic transmission .
Temporal Effects in Laboratory Settings
Over time, the effects of this compound can change in laboratory settings. For instance, it has been observed that small this compound oligomers of size 1–2 nm induce the strongest immune response in mice .
Dosage Effects in Animal Models
The effects of this compound vary with different dosages in animal models. It has been suggested that a “hormetic effect” exists in Amyloid b-Protein roles: a positive effect in the optimal dose range and a negative effect either above or below the range .
Metabolic Pathways
This compound is involved in several metabolic pathways. It is generated from amyloid precursor protein through sequential cleavage of β- and γ-secretases .
Transport and Distribution
This compound is transported and distributed within cells and tissues. The blood-brain barrier (BBB) is a critical portal regulating the bidirectional transport of Amyloid b-Protein between blood and brain .
Subcellular Localization
It has been suggested that this compound accumulates more in the lysosome, which could lead to its higher degradation and/or aggregation at lower lysosomal pH .
Preparation Methods
Synthetic Routes and Reaction Conditions: The preparation of Amyloid b-Protein (17-40) Ammonium typically involves solid-phase peptide synthesis. This method allows for the sequential addition of amino acids to a growing peptide chain anchored to a solid resin. The process includes:
Coupling: Each amino acid is activated and coupled to the resin-bound peptide.
Deprotection: Temporary protecting groups on the amino acids are removed to allow for the next coupling step.
Cleavage: The completed peptide is cleaved from the resin and purified.
Industrial Production Methods: Industrial production of this compound follows similar principles but on a larger scale. Automated peptide synthesizers and high-throughput purification systems are employed to increase efficiency and yield.
Types of Reactions:
Oxidation: this compound can undergo oxidation, particularly at methionine residues, leading to the formation of methionine sulfoxide.
Reduction: Reduction reactions can reverse oxidation, restoring methionine residues to their original state.
Substitution: Amino acid residues within the peptide can be substituted to study the effects on peptide structure and function.
Common Reagents and Conditions:
Oxidizing Agents: Hydrogen peroxide or other peroxides.
Reducing Agents: Dithiothreitol or other thiol-based reducing agents.
Substitution Reagents: Amino acid derivatives and coupling reagents like HBTU or HATU.
Major Products:
Oxidized Peptides: Methionine sulfoxide-containing peptides.
Reduced Peptides: Restored methionine residues.
Substituted Peptides: Variants of the original peptide with different amino acid compositions.
Scientific Research Applications
Amyloid b-Protein (17-40) Ammonium is extensively used in scientific research, particularly in the fields of:
Chemistry: Studying peptide synthesis, structure, and reactivity.
Biology: Investigating protein aggregation and its role in neurodegenerative diseases.
Medicine: Developing diagnostic tools and therapeutic strategies for Alzheimer’s disease.
Industry: Creating amyloid-based biomaterials for various applications, including sensors and drug delivery systems
Comparison with Similar Compounds
Amyloid b-Protein (1-40): Another fragment of the amyloid precursor protein, differing by the inclusion of the first 16 amino acids.
Amyloid b-Protein (1-42): A longer variant that is more prone to aggregation and is considered more toxic.
Uniqueness: Amyloid b-Protein (17-40) Ammonium is unique due to its specific sequence, which influences its aggregation properties and interactions with other molecules. This makes it a valuable tool for studying the specific mechanisms of amyloid formation and toxicity .
Properties
IUPAC Name |
(4S)-5-[[(2S)-1-[[(2S)-1-[[2-[[(2S)-1-[[(2S)-4-amino-1-[[(2S)-6-amino-1-[[2-[[(2S)-1-[[(2S,3S)-1-[[(2S,3S)-1-[[2-[[(2S)-1-[[(2S)-1-[[(2S)-1-[[2-[[2-[[(2S)-1-[[(1S)-1-carboxy-2-methylpropyl]amino]-3-methyl-1-oxobutan-2-yl]amino]-2-oxoethyl]amino]-2-oxoethyl]amino]-3-methyl-1-oxobutan-2-yl]amino]-4-methylsulfanyl-1-oxobutan-2-yl]amino]-4-methyl-1-oxopentan-2-yl]amino]-2-oxoethyl]amino]-3-methyl-1-oxopentan-2-yl]amino]-3-methyl-1-oxopentan-2-yl]amino]-1-oxopropan-2-yl]amino]-2-oxoethyl]amino]-1-oxohexan-2-yl]amino]-1,4-dioxobutan-2-yl]amino]-3-hydroxy-1-oxopropan-2-yl]amino]-2-oxoethyl]amino]-3-methyl-1-oxobutan-2-yl]amino]-3-carboxy-1-oxopropan-2-yl]amino]-4-[[(2S)-2-[[(2S)-2-[[(2S)-2-[[(2S)-2-[[(2S)-2-amino-4-methylpentanoyl]amino]-3-methylbutanoyl]amino]-3-phenylpropanoyl]amino]-3-phenylpropanoyl]amino]propanoyl]amino]-5-oxopentanoic acid | |
---|---|---|
Details | Computed by Lexichem TK 2.7.0 (PubChem release 2021.05.07) | |
Source | PubChem | |
URL | https://pubchem.ncbi.nlm.nih.gov | |
Description | Data deposited in or computed by PubChem | |
InChI |
InChI=1S/C110H178N26O31S/c1-22-61(17)90(106(162)118-51-80(141)121-71(43-55(5)6)99(155)125-70(39-41-168-21)97(153)132-85(56(7)8)104(160)116-48-78(139)114-49-82(143)130-87(58(11)12)108(164)134-89(60(15)16)110(166)167)136-109(165)91(62(18)23-2)135-93(149)63(19)119-79(140)50-115-95(151)68(36-30-31-40-111)124-101(157)74(46-77(113)138)127-103(159)76(53-137)122-81(142)52-117-105(161)86(57(9)10)133-102(158)75(47-84(146)147)128-96(152)69(37-38-83(144)145)123-92(148)64(20)120-98(154)72(44-65-32-26-24-27-33-65)126-100(156)73(45-66-34-28-25-29-35-66)129-107(163)88(59(13)14)131-94(150)67(112)42-54(3)4/h24-29,32-35,54-64,67-76,85-91,137H,22-23,30-31,36-53,111-112H2,1-21H3,(H2,113,138)(H,114,139)(H,115,151)(H,116,160)(H,117,161)(H,118,162)(H,119,140)(H,120,154)(H,121,141)(H,122,142)(H,123,148)(H,124,157)(H,125,155)(H,126,156)(H,127,159)(H,128,152)(H,129,163)(H,130,143)(H,131,150)(H,132,153)(H,133,158)(H,134,164)(H,135,149)(H,136,165)(H,144,145)(H,146,147)(H,166,167)/t61-,62-,63-,64-,67-,68-,69-,70-,71-,72-,73-,74-,75-,76-,85-,86-,87-,88-,89-,90-,91-/m0/s1 | |
Details | Computed by InChI 1.0.6 (PubChem release 2021.05.07) | |
Source | PubChem | |
URL | https://pubchem.ncbi.nlm.nih.gov | |
Description | Data deposited in or computed by PubChem | |
InChI Key |
QKAFHCYMOBODRG-FZSVSODXSA-N | |
Details | Computed by InChI 1.0.6 (PubChem release 2021.05.07) | |
Source | PubChem | |
URL | https://pubchem.ncbi.nlm.nih.gov | |
Description | Data deposited in or computed by PubChem | |
Canonical SMILES |
CCC(C)C(C(=O)NC(C(C)CC)C(=O)NCC(=O)NC(CC(C)C)C(=O)NC(CCSC)C(=O)NC(C(C)C)C(=O)NCC(=O)NCC(=O)NC(C(C)C)C(=O)NC(C(C)C)C(=O)O)NC(=O)C(C)NC(=O)CNC(=O)C(CCCCN)NC(=O)C(CC(=O)N)NC(=O)C(CO)NC(=O)CNC(=O)C(C(C)C)NC(=O)C(CC(=O)O)NC(=O)C(CCC(=O)O)NC(=O)C(C)NC(=O)C(CC1=CC=CC=C1)NC(=O)C(CC2=CC=CC=C2)NC(=O)C(C(C)C)NC(=O)C(CC(C)C)N | |
Details | Computed by OEChem 2.3.0 (PubChem release 2021.05.07) | |
Source | PubChem | |
URL | https://pubchem.ncbi.nlm.nih.gov | |
Description | Data deposited in or computed by PubChem | |
Isomeric SMILES |
CC[C@H](C)[C@@H](C(=O)N[C@@H]([C@@H](C)CC)C(=O)NCC(=O)N[C@@H](CC(C)C)C(=O)N[C@@H](CCSC)C(=O)N[C@@H](C(C)C)C(=O)NCC(=O)NCC(=O)N[C@@H](C(C)C)C(=O)N[C@@H](C(C)C)C(=O)O)NC(=O)[C@H](C)NC(=O)CNC(=O)[C@H](CCCCN)NC(=O)[C@H](CC(=O)N)NC(=O)[C@H](CO)NC(=O)CNC(=O)[C@H](C(C)C)NC(=O)[C@H](CC(=O)O)NC(=O)[C@H](CCC(=O)O)NC(=O)[C@H](C)NC(=O)[C@H](CC1=CC=CC=C1)NC(=O)[C@H](CC2=CC=CC=C2)NC(=O)[C@H](C(C)C)NC(=O)[C@H](CC(C)C)N | |
Details | Computed by OEChem 2.3.0 (PubChem release 2021.05.07) | |
Source | PubChem | |
URL | https://pubchem.ncbi.nlm.nih.gov | |
Description | Data deposited in or computed by PubChem | |
Molecular Formula |
C110H178N26O31S | |
Details | Computed by PubChem 2.1 (PubChem release 2021.05.07) | |
Source | PubChem | |
URL | https://pubchem.ncbi.nlm.nih.gov | |
Description | Data deposited in or computed by PubChem | |
Molecular Weight |
2392.8 g/mol | |
Details | Computed by PubChem 2.1 (PubChem release 2021.05.07) | |
Source | PubChem | |
URL | https://pubchem.ncbi.nlm.nih.gov | |
Description | Data deposited in or computed by PubChem | |
Disclaimer and Information on In-Vitro Research Products
Please be aware that all articles and product information presented on BenchChem are intended solely for informational purposes. The products available for purchase on BenchChem are specifically designed for in-vitro studies, which are conducted outside of living organisms. In-vitro studies, derived from the Latin term "in glass," involve experiments performed in controlled laboratory settings using cells or tissues. It is important to note that these products are not categorized as medicines or drugs, and they have not received approval from the FDA for the prevention, treatment, or cure of any medical condition, ailment, or disease. We must emphasize that any form of bodily introduction of these products into humans or animals is strictly prohibited by law. It is essential to adhere to these guidelines to ensure compliance with legal and ethical standards in research and experimentation.