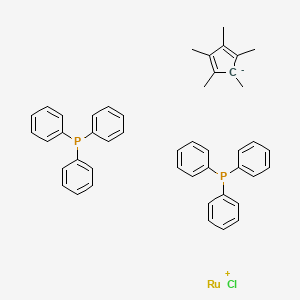
Cp*RuCl(PPh3)2
Overview
Description
Pentamethylcyclopentadienylbis(triphenylphosphine)ruthenium(II) chloride, commonly known as Cp*RuCl(PPh3)2, is an organometallic compound with the formula [(C10H15)Ru(P(C6H5)3)2Cl]. This compound is a half-sandwich complex featuring a ruthenium center coordinated to a pentamethylcyclopentadienyl ligand, two triphenylphosphine ligands, and a chloride ligand. It is widely used as a catalyst in various organic transformations due to its stability and reactivity .
Mechanism of Action
Target of Action
It is known that this compound is used in a variety of organometallic synthetic and catalytic transformations .
Mode of Action
Cp*RuCl(PPh3)2 undergoes a variety of reactions often involving substitution of the chloride . For instance, with phenylacetylene, it gives the phenyl vinylidene complex . Displacement of one PPh3 by carbon monoxide affords a chiral compound . These reactions indicate that the compound interacts with its targets through a process of ligand exchange.
Biochemical Pathways
It is known that this compound serves as a catalyst for a variety of specialized reactions . For example, in the presence of NH4PF6, it catalyzes the isomerization of allylic alcohols to the corresponding saturated carbonyls .
Pharmacokinetics
It is known that this compound is an air-stable orange crystalline solid and is soluble in chloroform, dichloromethane, and acetone , which may influence its bioavailability.
Result of Action
It is known that this compound is used in a variety of organometallic synthetic and catalytic transformations , indicating that it can induce significant changes at the molecular level.
Action Environment
It is known that this compound is air-stable , suggesting that it is relatively resistant to degradation in the presence of oxygen.
Biochemical Analysis
Biochemical Properties
It is known to be a catalyst employed in the cycloaddition of azides with acetylenes, producing exclusively the 1,5-disubstitutedtriazoles . This suggests that it may interact with enzymes, proteins, and other biomolecules involved in these reactions.
Molecular Mechanism
It is known to undergo a variety of reactions often by involving substitution of the chloride . For example, with phenylacetylene it gives the phenyl vinylidene complex
Preparation Methods
Synthetic Routes and Reaction Conditions
Pentamethylcyclopentadienylbis(triphenylphosphine)ruthenium(II) chloride can be synthesized by reacting ruthenium(III) chloride with triphenylphosphine and pentamethylcyclopentadiene in ethanol. The reaction typically involves heating the mixture to facilitate the formation of the desired complex .
Industrial Production Methods
While specific industrial production methods for Cp*RuCl(PPh3)2 are not extensively documented, the synthesis generally follows similar principles as laboratory preparation, with adjustments for scale and efficiency. The use of high-purity reagents and controlled reaction conditions ensures the production of high-quality catalysts for industrial applications.
Chemical Reactions Analysis
Types of Reactions
Pentamethylcyclopentadienylbis(triphenylphosphine)ruthenium(II) chloride undergoes various types of reactions, including:
Substitution Reactions: The chloride ligand can be substituted by other ligands such as carbon monoxide or hydride.
Cycloaddition Reactions: It catalyzes the cycloaddition of azides with acetylenes, producing 1,5-disubstituted 1,2,3-triazoles.
Polymerization Reactions: It acts as a catalyst in living radical polymerization.
Common Reagents and Conditions
Phenylacetylene: Used in cycloaddition reactions to form phenyl vinylidene complexes.
Carbon Monoxide: Used in substitution reactions to form chiral compounds.
Azides: React with acetylenes in the presence of Cp*RuCl(PPh3)2 to form triazoles.
Major Products Formed
1,5-Disubstituted 1,2,3-Triazoles: Formed from the cycloaddition of azides and acetylenes.
Phenyl Vinylidene Complexes: Formed from the reaction with phenylacetylene.
Chiral Compounds: Formed from substitution reactions with carbon monoxide.
Scientific Research Applications
Pentamethylcyclopentadienylbis(triphenylphosphine)ruthenium(II) chloride has a wide range of applications in scientific research:
Comparison with Similar Compounds
Similar Compounds
Chloro(cyclopentadienyl)bis(triphenylphosphine)ruthenium: Similar structure but with a cyclopentadienyl ligand instead of a pentamethylcyclopentadienyl ligand.
Dichlorotris(triphenylphosphine)ruthenium(II): Contains three triphenylphosphine ligands and two chloride ligands.
Uniqueness
Pentamethylcyclopentadienylbis(triphenylphosphine)ruthenium(II) chloride is unique due to its pentamethylcyclopentadienyl ligand, which provides enhanced stability and reactivity compared to similar compounds with cyclopentadienyl ligands. This makes it particularly effective in catalyzing regioselective reactions and polymerizations .
Properties
IUPAC Name |
chlororuthenium(1+);1,2,3,4,5-pentamethylcyclopenta-1,3-diene;triphenylphosphane | |
---|---|---|
Details | Computed by Lexichem TK 2.7.0 (PubChem release 2021.05.07) | |
Source | PubChem | |
URL | https://pubchem.ncbi.nlm.nih.gov | |
Description | Data deposited in or computed by PubChem | |
InChI |
InChI=1S/2C18H15P.C10H15.ClH.Ru/c2*1-4-10-16(11-5-1)19(17-12-6-2-7-13-17)18-14-8-3-9-15-18;1-6-7(2)9(4)10(5)8(6)3;;/h2*1-15H;1-5H3;1H;/q;;-1;;+2/p-1 | |
Details | Computed by InChI 1.0.6 (PubChem release 2021.05.07) | |
Source | PubChem | |
URL | https://pubchem.ncbi.nlm.nih.gov | |
Description | Data deposited in or computed by PubChem | |
InChI Key |
AHWRJPOOFGXEKF-UHFFFAOYSA-M | |
Details | Computed by InChI 1.0.6 (PubChem release 2021.05.07) | |
Source | PubChem | |
URL | https://pubchem.ncbi.nlm.nih.gov | |
Description | Data deposited in or computed by PubChem | |
Canonical SMILES |
C[C-]1C(=C(C(=C1C)C)C)C.C1=CC=C(C=C1)P(C2=CC=CC=C2)C3=CC=CC=C3.C1=CC=C(C=C1)P(C2=CC=CC=C2)C3=CC=CC=C3.Cl[Ru+] | |
Details | Computed by OEChem 2.3.0 (PubChem release 2021.05.07) | |
Source | PubChem | |
URL | https://pubchem.ncbi.nlm.nih.gov | |
Description | Data deposited in or computed by PubChem | |
Molecular Formula |
C46H45ClP2Ru | |
Details | Computed by PubChem 2.1 (PubChem release 2021.05.07) | |
Source | PubChem | |
URL | https://pubchem.ncbi.nlm.nih.gov | |
Description | Data deposited in or computed by PubChem | |
DSSTOX Substance ID |
DTXSID00746198 | |
Record name | Chlororuthenium(1+) 1,2,3,4,5-pentamethylcyclopenta-2,4-dien-1-ide--triphenylphosphane (1/1/2) | |
Source | EPA DSSTox | |
URL | https://comptox.epa.gov/dashboard/DTXSID00746198 | |
Description | DSSTox provides a high quality public chemistry resource for supporting improved predictive toxicology. | |
Molecular Weight |
796.3 g/mol | |
Details | Computed by PubChem 2.1 (PubChem release 2021.05.07) | |
Source | PubChem | |
URL | https://pubchem.ncbi.nlm.nih.gov | |
Description | Data deposited in or computed by PubChem | |
CAS No. |
92361-49-4 | |
Record name | Chlororuthenium(1+) 1,2,3,4,5-pentamethylcyclopenta-2,4-dien-1-ide--triphenylphosphane (1/1/2) | |
Source | EPA DSSTox | |
URL | https://comptox.epa.gov/dashboard/DTXSID00746198 | |
Description | DSSTox provides a high quality public chemistry resource for supporting improved predictive toxicology. | |
Disclaimer and Information on In-Vitro Research Products
Please be aware that all articles and product information presented on BenchChem are intended solely for informational purposes. The products available for purchase on BenchChem are specifically designed for in-vitro studies, which are conducted outside of living organisms. In-vitro studies, derived from the Latin term "in glass," involve experiments performed in controlled laboratory settings using cells or tissues. It is important to note that these products are not categorized as medicines or drugs, and they have not received approval from the FDA for the prevention, treatment, or cure of any medical condition, ailment, or disease. We must emphasize that any form of bodily introduction of these products into humans or animals is strictly prohibited by law. It is essential to adhere to these guidelines to ensure compliance with legal and ethical standards in research and experimentation.