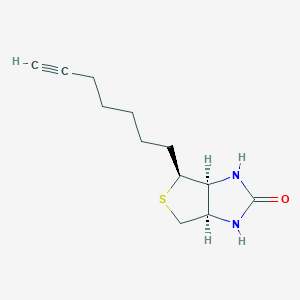
DecarboxyBiotin-Alkyne
- Click on QUICK INQUIRY to receive a quote from our team of experts.
- With the quality product at a COMPETITIVE price, you can focus more on your research.
Overview
Description
DecarboxyBiotin-Alkyne is a biotinylated alkyne compound that is used in various scientific research applications. It is a highly reactive compound that can be used to study protein-protein interactions, protein-DNA interactions, and other biochemical and physiological processes. DecarboxyBiotin-Alkyne has a number of advantages over other biotinylation methods, including its high reactivity, its ability to be used in a variety of applications, and its low cost.
Scientific Research Applications
Biosynthesis of Natural Products
DecarboxyBiotin-Alkyne: is instrumental in the biosynthesis of alkyne-containing natural products. Researchers have been inspired by the advantages of acetylenic products in various fields and have focused on discovering biosynthetic enzymes and pathways for alkyne formation . This has led to the development of de novo biosynthetic strategies for alkyne-tagged compound production.
Mechanism of Action
Target of Action
DecarboxyBiotin-Alkyne is a click chemistry reagent . Its primary targets are nucleic acids, lipids, proteins, and other molecules . Click chemistry is a powerful tool for binding these molecules together, and it has been used in many research fields due to its beneficial characteristics .
Mode of Action
DecarboxyBiotin-Alkyne interacts with its targets through a process known as click chemistry . This process is characterized by high yield, high specificity, and simplicity . The compound contains an azide group, which is a key component in click chemistry reactions . The azide group reacts with alkynes to form a stable triazole ring, a process that is highly selective and efficient .
Biochemical Pathways
It is known that the compound plays a role in thebiosynthesis of alkyne-containing natural products . These natural products are widely distributed in microbes and plants . The compound’s ability to bind various molecules suggests that it may affect multiple biochemical pathways .
Result of Action
The result of DecarboxyBiotin-Alkyne’s action is the formation of stable connections between various biological molecules . This can lead to the creation of complex structures and networks, which can have various effects at the molecular and cellular levels .
Action Environment
The action of DecarboxyBiotin-Alkyne can be influenced by various environmental factors. For instance, the efficiency of click chemistry reactions can be affected by factors such as temperature, pH, and the presence of catalysts . Click chemistry is generally considered to be robust and reliable under a wide range of conditions .
properties
IUPAC Name |
(3aS,4S,6aR)-4-hept-6-ynyl-1,3,3a,4,6,6a-hexahydrothieno[3,4-d]imidazol-2-one |
Source
|
---|---|---|
Details | Computed by LexiChem 2.6.6 (PubChem release 2019.06.18) | |
Source | PubChem | |
URL | https://pubchem.ncbi.nlm.nih.gov | |
Description | Data deposited in or computed by PubChem | |
InChI |
InChI=1S/C12H18N2OS/c1-2-3-4-5-6-7-10-11-9(8-16-10)13-12(15)14-11/h1,9-11H,3-8H2,(H2,13,14,15)/t9-,10-,11-/m0/s1 |
Source
|
Details | Computed by InChI 1.0.5 (PubChem release 2019.06.18) | |
Source | PubChem | |
URL | https://pubchem.ncbi.nlm.nih.gov | |
Description | Data deposited in or computed by PubChem | |
InChI Key |
HWFZBYFQQMRYQB-DCAQKATOSA-N |
Source
|
Details | Computed by InChI 1.0.5 (PubChem release 2019.06.18) | |
Source | PubChem | |
URL | https://pubchem.ncbi.nlm.nih.gov | |
Description | Data deposited in or computed by PubChem | |
Canonical SMILES |
C#CCCCCCC1C2C(CS1)NC(=O)N2 |
Source
|
Details | Computed by OEChem 2.1.5 (PubChem release 2019.06.18) | |
Source | PubChem | |
URL | https://pubchem.ncbi.nlm.nih.gov | |
Description | Data deposited in or computed by PubChem | |
Isomeric SMILES |
C#CCCCCC[C@H]1[C@@H]2[C@H](CS1)NC(=O)N2 |
Source
|
Details | Computed by OEChem 2.1.5 (PubChem release 2019.06.18) | |
Source | PubChem | |
URL | https://pubchem.ncbi.nlm.nih.gov | |
Description | Data deposited in or computed by PubChem | |
Molecular Formula |
C12H18N2OS |
Source
|
Details | Computed by PubChem 2.1 (PubChem release 2019.06.18) | |
Source | PubChem | |
URL | https://pubchem.ncbi.nlm.nih.gov | |
Description | Data deposited in or computed by PubChem | |
Molecular Weight |
238.35 g/mol |
Source
|
Details | Computed by PubChem 2.1 (PubChem release 2021.05.07) | |
Source | PubChem | |
URL | https://pubchem.ncbi.nlm.nih.gov | |
Description | Data deposited in or computed by PubChem | |
Product Name |
DecarboxyBiotin-Alkyne |
Disclaimer and Information on In-Vitro Research Products
Please be aware that all articles and product information presented on BenchChem are intended solely for informational purposes. The products available for purchase on BenchChem are specifically designed for in-vitro studies, which are conducted outside of living organisms. In-vitro studies, derived from the Latin term "in glass," involve experiments performed in controlled laboratory settings using cells or tissues. It is important to note that these products are not categorized as medicines or drugs, and they have not received approval from the FDA for the prevention, treatment, or cure of any medical condition, ailment, or disease. We must emphasize that any form of bodily introduction of these products into humans or animals is strictly prohibited by law. It is essential to adhere to these guidelines to ensure compliance with legal and ethical standards in research and experimentation.