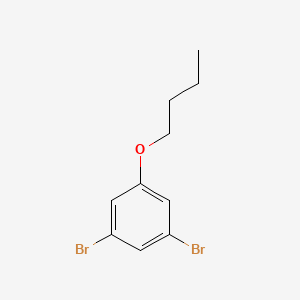
1,3-Dibromo-5-butoxybenzene
Overview
Description
1,3-Dibromo-5-butoxybenzene is a chemical compound with the molecular formula C₁₀H₁₂Br₂O. It is known for its unique physical and chemical properties, making it significant in various research and industrial applications. This compound is utilized in fields such as pharmaceuticals, agriculture, and material science due to its reactivity and stability.
Scientific Research Applications
1,3-Dibromo-5-butoxybenzene is widely used in scientific research due to its versatility:
Chemistry: It serves as an intermediate in the synthesis of complex organic molecules.
Biology: The compound is used in the study of enzyme inhibition and protein interactions.
Industry: Utilized in the production of polymers, resins, and other materials.
Safety and Hazards
While specific safety and hazard information for 1,3-Dibromo-5-butoxybenzene is not available, similar compounds like 1,3-dibromobenzene are considered hazardous by the 2012 OSHA Hazard Communication Standard (29 CFR 1910.1200). They are classified as flammable liquids, skin corrosive/irritant, serious eye damage/eye irritant, and specific target organ toxicity (single exposure) with the target organ being the respiratory system .
Mechanism of Action
Mode of Action
. These reactions can lead to changes in the molecular structure of the target, potentially altering its function.
Biochemical Pathways
They can cause modifications in proteins and nucleic acids, potentially affecting processes such as signal transduction, gene expression, and enzymatic activity .
Pharmacokinetics
Factors such as solubility, stability, and molecular size can influence how a compound is absorbed and distributed in the body, how it is metabolized, and how it is excreted .
Action Environment
Environmental factors can significantly influence the action, efficacy, and stability of a compound. Factors such as temperature, pH, and the presence of other molecules can affect a compound’s reactivity and stability. For instance, certain reactions may only occur under specific conditions or in the presence of certain catalysts . .
Preparation Methods
Synthetic Routes and Reaction Conditions
1,3-Dibromo-5-butoxybenzene can be synthesized through the bromination of 5-butoxybenzene. The process typically involves the use of bromine or N-bromosuccinimide (NBS) as the brominating agents. The reaction is carried out in a solvent such as carbon tetrachloride (CCl₄) under reflux conditions. The presence of a catalyst like benzoyl peroxide can enhance the reaction efficiency .
Industrial Production Methods
In industrial settings, the production of this compound follows similar synthetic routes but on a larger scale. The use of continuous flow reactors and automated systems ensures consistent product quality and yield. The reaction conditions are optimized to minimize byproducts and maximize the efficiency of the bromination process .
Chemical Reactions Analysis
Types of Reactions
1,3-Dibromo-5-butoxybenzene undergoes various chemical reactions, including:
Substitution Reactions: The bromine atoms can be substituted with other functional groups through nucleophilic substitution reactions.
Oxidation Reactions: The compound can be oxidized to form corresponding quinones or other oxidized derivatives.
Reduction Reactions: Reduction of the bromine atoms can yield the corresponding butoxybenzene derivatives.
Common Reagents and Conditions
Nucleophilic Substitution: Common reagents include sodium hydroxide (NaOH) or potassium hydroxide (KOH) in polar solvents.
Oxidation: Reagents such as potassium permanganate (KMnO₄) or chromium trioxide (CrO₃) are used under acidic conditions.
Reduction: Reducing agents like lithium aluminum hydride (LiAlH₄) or sodium borohydride (NaBH₄) are employed.
Major Products Formed
Substitution: Formation of various substituted benzene derivatives.
Oxidation: Production of quinones and other oxidized compounds.
Reduction: Generation of butoxybenzene derivatives.
Comparison with Similar Compounds
Similar Compounds
1,3-Dibromo-5,5-dimethylhydantoin: A brominating agent with similar reactivity but different applications.
1,3-Dibromo-5,5-dimethylhydantoin: Used in bromination reactions and as a disinfectant.
Uniqueness
1,3-Dibromo-5-butoxybenzene is unique due to its butoxy group, which imparts distinct physical and chemical properties. This makes it more suitable for specific applications in organic synthesis and material science compared to other dibromo compounds .
Properties
IUPAC Name |
1,3-dibromo-5-butoxybenzene | |
---|---|---|
Details | Computed by Lexichem TK 2.7.0 (PubChem release 2021.05.07) | |
Source | PubChem | |
URL | https://pubchem.ncbi.nlm.nih.gov | |
Description | Data deposited in or computed by PubChem | |
InChI |
InChI=1S/C10H12Br2O/c1-2-3-4-13-10-6-8(11)5-9(12)7-10/h5-7H,2-4H2,1H3 | |
Details | Computed by InChI 1.0.6 (PubChem release 2021.05.07) | |
Source | PubChem | |
URL | https://pubchem.ncbi.nlm.nih.gov | |
Description | Data deposited in or computed by PubChem | |
InChI Key |
UIJOUNUFUONLQL-UHFFFAOYSA-N | |
Details | Computed by InChI 1.0.6 (PubChem release 2021.05.07) | |
Source | PubChem | |
URL | https://pubchem.ncbi.nlm.nih.gov | |
Description | Data deposited in or computed by PubChem | |
Canonical SMILES |
CCCCOC1=CC(=CC(=C1)Br)Br | |
Details | Computed by OEChem 2.3.0 (PubChem release 2021.05.07) | |
Source | PubChem | |
URL | https://pubchem.ncbi.nlm.nih.gov | |
Description | Data deposited in or computed by PubChem | |
Molecular Formula |
C10H12Br2O | |
Details | Computed by PubChem 2.1 (PubChem release 2021.05.07) | |
Source | PubChem | |
URL | https://pubchem.ncbi.nlm.nih.gov | |
Description | Data deposited in or computed by PubChem | |
Molecular Weight |
308.01 g/mol | |
Details | Computed by PubChem 2.1 (PubChem release 2021.05.07) | |
Source | PubChem | |
URL | https://pubchem.ncbi.nlm.nih.gov | |
Description | Data deposited in or computed by PubChem | |
Retrosynthesis Analysis
AI-Powered Synthesis Planning: Our tool employs the Template_relevance Pistachio, Template_relevance Bkms_metabolic, Template_relevance Pistachio_ringbreaker, Template_relevance Reaxys, Template_relevance Reaxys_biocatalysis model, leveraging a vast database of chemical reactions to predict feasible synthetic routes.
One-Step Synthesis Focus: Specifically designed for one-step synthesis, it provides concise and direct routes for your target compounds, streamlining the synthesis process.
Accurate Predictions: Utilizing the extensive PISTACHIO, BKMS_METABOLIC, PISTACHIO_RINGBREAKER, REAXYS, REAXYS_BIOCATALYSIS database, our tool offers high-accuracy predictions, reflecting the latest in chemical research and data.
Strategy Settings
Precursor scoring | Relevance Heuristic |
---|---|
Min. plausibility | 0.01 |
Model | Template_relevance |
Template Set | Pistachio/Bkms_metabolic/Pistachio_ringbreaker/Reaxys/Reaxys_biocatalysis |
Top-N result to add to graph | 6 |
Feasible Synthetic Routes
Disclaimer and Information on In-Vitro Research Products
Please be aware that all articles and product information presented on BenchChem are intended solely for informational purposes. The products available for purchase on BenchChem are specifically designed for in-vitro studies, which are conducted outside of living organisms. In-vitro studies, derived from the Latin term "in glass," involve experiments performed in controlled laboratory settings using cells or tissues. It is important to note that these products are not categorized as medicines or drugs, and they have not received approval from the FDA for the prevention, treatment, or cure of any medical condition, ailment, or disease. We must emphasize that any form of bodily introduction of these products into humans or animals is strictly prohibited by law. It is essential to adhere to these guidelines to ensure compliance with legal and ethical standards in research and experimentation.