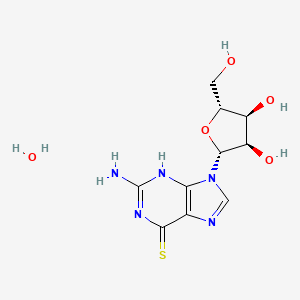
Thioguanosine hydrate
- Click on QUICK INQUIRY to receive a quote from our team of experts.
- With the quality product at a COMPETITIVE price, you can focus more on your research.
Overview
Description
Thioguanosine hydrate is a purine nucleoside analog, specifically a derivative of guanosine where the oxygen atom at the sixth position is replaced by a sulfur atom. This compound is known for its potential therapeutic applications, particularly in oncology and virology. It is characterized by its yellowish-beige powder form and is hygroscopic, meaning it readily absorbs moisture from the environment .
Mechanism of Action
Target of Action
The primary target of 6-Thioguanosine hydrate is the enzyme hypoxanthine-guanine phosphoribosyltransferase (HGPRTase) . This enzyme plays a crucial role in the purine salvage pathway, which is essential for nucleotide synthesis .
Mode of Action
6-Thioguanosine hydrate competes with hypoxanthine and guanine for HGPRTase and is converted into 6-thioguanilyic acid (TGMP), which reaches high intracellular concentrations at therapeutic doses . The biologically active compounds are deoxy-thioguanosine triphosphate (TdGTP) and thioguanosine triphosphate (TGTP) . TdGTP is incorporated into DNA, which ultimately causes DNA strand breaks due to faulty repair and perturbs RNA transcription .
Biochemical Pathways
6-Thioguanosine hydrate affects the purine salvage pathway . This pathway is responsible for the synthesis of nucleotides from intermediates in the degradative pathway for nucleotides. The disruption of this pathway by 6-Thioguanosine hydrate leads to the accumulation of TGMP, which inhibits the conversion of inosinic acid to xanthylic acid .
Pharmacokinetics
It is known that the compound is metabolized intracellularly . The activation of 6-Thioguanosine hydrate requires only the enzyme HGPRT, which catalyzes its conversion to 6-thioguanosine monophosphate (6-TGMP) .
Result of Action
The molecular and cellular effects of 6-Thioguanosine hydrate’s action are primarily due to its incorporation into DNA. This results in DNA strand breaks due to faulty repair and perturbation of RNA transcription . These disruptions can lead to cell death, making 6-Thioguanosine hydrate effective against certain types of cancer .
Action Environment
The action, efficacy, and stability of 6-Thioguanosine hydrate can be influenced by various environmental factors. For instance, exposure to UVA radiation has been shown to induce DNA damage and cell death . Additionally, the compound’s cytotoxicity can be modulated by N9-glycosylation
Biochemical Analysis
Biochemical Properties
6-Thioguanosine hydrate plays a significant role in biochemical reactions. It is metabolically activated by the enzyme hypoxanthine-guanine phosphoribosyl transferase (HGPRT) to form 6-thioguanosine monophosphate (6-TGMP) . The biologically active compounds are deoxy-thioguanosine triphosphate (TdGTP) and thioguanosine triphosphate (TGTP) .
Cellular Effects
6-Thioguanosine hydrate has been shown to have significant effects on various types of cells and cellular processes. It exhibits a delayed cytotoxic activity, halting the cell cycle in the G2 phase after one round of replication . It has also been shown to have antiproliferative effects on cells that are resistant to thiopurines .
Molecular Mechanism
The mechanism of action of 6-Thioguanosine hydrate involves its conversion to 6-thioguanosine monophosphate (6-TGMP) by the enzyme HGPRT . The biologically active compounds, TdGTP and TGTP, are incorporated into DNA, which ultimately causes DNA strand breaks due to faulty repair and perturbs RNA transcription .
Temporal Effects in Laboratory Settings
The effects of 6-Thioguanosine hydrate change over time in laboratory settings. It has been shown that the presence of 6-Thioguanosine hydrate diminishes the thermodynamic stability of RNA duplexes . When placed at a dangling end, a 6-Thioguanosine residue actually exerts a weak stabilizing effect .
Dosage Effects in Animal Models
In animal models, the effects of 6-Thioguanosine hydrate vary with different dosages. For instance, in a study involving diabetic mice, the mice received 6-Thioguanosine hydrate intravitreally at doses of 0.5–1–2.5 mg/kg .
Metabolic Pathways
6-Thioguanosine hydrate is involved in the purine salvage pathway. It is converted to 6-thioguanosine monophosphate (6-TGMP) by the enzyme HGPRT . The biologically active compounds, TdGTP and TGTP, are then formed, which are incorporated into DNA and RNA, respectively .
Transport and Distribution
It is known that it is metabolically activated and incorporated into DNA and RNA, suggesting that it can be transported across cellular compartments .
Subcellular Localization
The subcellular localization of 6-Thioguanosine hydrate is not well-defined. Given its incorporation into DNA and RNA, it can be inferred that it is localized in the nucleus and cytoplasm where these molecules are present .
Preparation Methods
Thioguanosine hydrate can be synthesized through the thiation of guanine using phosphorus pentasulfide. This reaction involves the substitution of the oxygen atom in guanine with a sulfur atom to form thioguanine, which is then ribosylated to produce thioguanosine . Industrial production methods typically involve the use of advanced organic synthesis techniques to ensure high purity and yield.
Chemical Reactions Analysis
Thioguanosine hydrate undergoes various chemical reactions, including:
Oxidation: It can be oxidized to form disulfides, which are redox-responsive and can form hydrogels.
Reduction: The compound can be reduced under specific conditions, leading to the formation of guanosine.
Substitution: Thioguanosine can participate in substitution reactions, particularly in the presence of nucleophiles.
Common reagents used in these reactions include oxidizing agents like hydrogen peroxide and reducing agents such as sodium borohydride. The major products formed from these reactions include disulfides and guanosine derivatives .
Scientific Research Applications
Thioguanosine hydrate has a wide range of applications in scientific research:
Chemistry: It is used in the study of nucleoside analogs and their reactivity with various chemical species.
Biology: The compound is employed in the investigation of nucleic acid interactions and modifications.
Industry: It is used in the development of redox-responsive materials and hydrogels for various applications.
Comparison with Similar Compounds
Thioguanosine hydrate is unique among nucleoside analogs due to its sulfur atom substitution, which imparts distinct chemical and biological properties. Similar compounds include:
6-Thioguanine: Another purine analog used in cancer treatment.
8-Thioguanosine: Known for its redox-responsive properties and applications in hydrogel formation.
6-Thioguanosine Monophosphate: A derivative used in the study of thiopurine resistance in cancer cells.
This compound stands out due to its specific reactivity and potential therapeutic applications, making it a valuable compound in both research and clinical settings.
Properties
IUPAC Name |
2-amino-9-[(2R,3R,4S,5R)-3,4-dihydroxy-5-(hydroxymethyl)oxolan-2-yl]-3H-purine-6-thione;hydrate |
Source
|
---|---|---|
Details | Computed by Lexichem TK 2.7.0 (PubChem release 2021.05.07) | |
Source | PubChem | |
URL | https://pubchem.ncbi.nlm.nih.gov | |
Description | Data deposited in or computed by PubChem | |
InChI |
InChI=1S/C10H13N5O4S.H2O/c11-10-13-7-4(8(20)14-10)12-2-15(7)9-6(18)5(17)3(1-16)19-9;/h2-3,5-6,9,16-18H,1H2,(H3,11,13,14,20);1H2/t3-,5-,6-,9-;/m1./s1 |
Source
|
Details | Computed by InChI 1.0.6 (PubChem release 2021.05.07) | |
Source | PubChem | |
URL | https://pubchem.ncbi.nlm.nih.gov | |
Description | Data deposited in or computed by PubChem | |
InChI Key |
CEMWFPKZUAKCRS-GWTDSMLYSA-N |
Source
|
Details | Computed by InChI 1.0.6 (PubChem release 2021.05.07) | |
Source | PubChem | |
URL | https://pubchem.ncbi.nlm.nih.gov | |
Description | Data deposited in or computed by PubChem | |
Canonical SMILES |
C1=NC2=C(N1C3C(C(C(O3)CO)O)O)NC(=NC2=S)N.O |
Source
|
Details | Computed by OEChem 2.3.0 (PubChem release 2021.05.07) | |
Source | PubChem | |
URL | https://pubchem.ncbi.nlm.nih.gov | |
Description | Data deposited in or computed by PubChem | |
Isomeric SMILES |
C1=NC2=C(N1[C@H]3[C@@H]([C@@H]([C@H](O3)CO)O)O)NC(=NC2=S)N.O |
Source
|
Details | Computed by OEChem 2.3.0 (PubChem release 2021.05.07) | |
Source | PubChem | |
URL | https://pubchem.ncbi.nlm.nih.gov | |
Description | Data deposited in or computed by PubChem | |
Molecular Formula |
C10H15N5O5S |
Source
|
Details | Computed by PubChem 2.1 (PubChem release 2021.05.07) | |
Source | PubChem | |
URL | https://pubchem.ncbi.nlm.nih.gov | |
Description | Data deposited in or computed by PubChem | |
Molecular Weight |
317.32 g/mol |
Source
|
Details | Computed by PubChem 2.1 (PubChem release 2021.05.07) | |
Source | PubChem | |
URL | https://pubchem.ncbi.nlm.nih.gov | |
Description | Data deposited in or computed by PubChem | |
Disclaimer and Information on In-Vitro Research Products
Please be aware that all articles and product information presented on BenchChem are intended solely for informational purposes. The products available for purchase on BenchChem are specifically designed for in-vitro studies, which are conducted outside of living organisms. In-vitro studies, derived from the Latin term "in glass," involve experiments performed in controlled laboratory settings using cells or tissues. It is important to note that these products are not categorized as medicines or drugs, and they have not received approval from the FDA for the prevention, treatment, or cure of any medical condition, ailment, or disease. We must emphasize that any form of bodily introduction of these products into humans or animals is strictly prohibited by law. It is essential to adhere to these guidelines to ensure compliance with legal and ethical standards in research and experimentation.