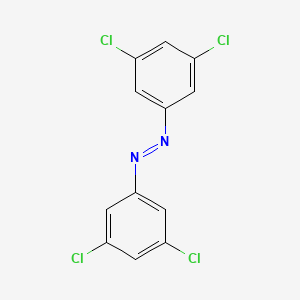
3,3',5,5'-Tetrachloroazobenzene
Overview
Description
3,3’,5,5’-Tetrachloroazobenzene is an organic compound belonging to the class of azobenzenes, characterized by the presence of two benzene rings connected by a nitrogen-nitrogen double bond The compound is distinguished by the substitution of chlorine atoms at the 3, 3’, 5, and 5’ positions on the benzene rings
Preparation Methods
Synthetic Routes and Reaction Conditions
The synthesis of 3,3’,5,5’-Tetrachloroazobenzene typically involves the reduction of 3,5-dichloronitrobenzene. One efficient method includes the use of lithium aluminum hydride as a reducing agent. The reaction proceeds under controlled conditions to ensure high purity and yield .
Industrial Production Methods
Industrial production of 3,3’,5,5’-Tetrachloroazobenzene may involve similar synthetic routes but on a larger scale. The process is optimized for efficiency, cost-effectiveness, and safety. The use of high-pressure liquid chromatography ensures the purity of the final product .
Chemical Reactions Analysis
Types of Reactions
3,3’,5,5’-Tetrachloroazobenzene undergoes various chemical reactions, including:
Oxidation: The compound can be oxidized to form corresponding azoxy and hydrazo derivatives.
Reduction: Reduction reactions can convert it back to its precursor compounds.
Substitution: Chlorine atoms can be substituted with other functional groups under specific conditions.
Common Reagents and Conditions
Oxidation: Common oxidizing agents include manganese dioxide.
Reduction: Lithium aluminum hydride is frequently used as a reducing agent.
Substitution: Reagents such as sodium hydroxide or other bases can facilitate substitution reactions.
Major Products Formed
The major products formed from these reactions include azoxy and hydrazo derivatives, which have their own unique properties and applications .
Scientific Research Applications
3,3’,5,5’-Tetrachloroazobenzene has diverse applications in scientific research:
Chemistry: Used as a reagent in organic synthesis and as a precursor for other chemical compounds.
Biology: Studied for its potential effects on biological systems, including its neurotoxic effects.
Medicine: Investigated for its potential therapeutic applications, although its toxicity limits its use.
Industry: Utilized in the production of dyes, pigments, and other industrial chemicals.
Mechanism of Action
The mechanism of action of 3,3’,5,5’-Tetrachloroazobenzene involves its interaction with biological molecules. It can disrupt normal cellular processes by interfering with enzyme activity and cellular signaling pathways. The compound’s structure allows it to bind to specific molecular targets, leading to various biological effects .
Comparison with Similar Compounds
Similar Compounds
3,3’,4,4’-Tetrachloroazobenzene: Similar in structure but with chlorine atoms at different positions.
3,3’,4,4’-Tetrachloroazoxybenzene: An oxidized derivative with an additional oxygen atom.
Uniqueness
3,3’,5,5’-Tetrachloroazobenzene is unique due to its specific substitution pattern, which influences its chemical reactivity and biological activity. This makes it distinct from other azobenzene derivatives and valuable for specific applications .
Properties
IUPAC Name |
bis(3,5-dichlorophenyl)diazene | |
---|---|---|
Details | Computed by Lexichem TK 2.7.0 (PubChem release 2021.05.07) | |
Source | PubChem | |
URL | https://pubchem.ncbi.nlm.nih.gov | |
Description | Data deposited in or computed by PubChem | |
InChI |
InChI=1S/C12H6Cl4N2/c13-7-1-8(14)4-11(3-7)17-18-12-5-9(15)2-10(16)6-12/h1-6H | |
Details | Computed by InChI 1.0.6 (PubChem release 2021.05.07) | |
Source | PubChem | |
URL | https://pubchem.ncbi.nlm.nih.gov | |
Description | Data deposited in or computed by PubChem | |
InChI Key |
CIAIFGDZDBTOCY-UHFFFAOYSA-N | |
Details | Computed by InChI 1.0.6 (PubChem release 2021.05.07) | |
Source | PubChem | |
URL | https://pubchem.ncbi.nlm.nih.gov | |
Description | Data deposited in or computed by PubChem | |
Canonical SMILES |
C1=C(C=C(C=C1Cl)Cl)N=NC2=CC(=CC(=C2)Cl)Cl | |
Details | Computed by OEChem 2.3.0 (PubChem release 2021.05.07) | |
Source | PubChem | |
URL | https://pubchem.ncbi.nlm.nih.gov | |
Description | Data deposited in or computed by PubChem | |
Molecular Formula |
C12H6Cl4N2 | |
Details | Computed by PubChem 2.1 (PubChem release 2021.05.07) | |
Source | PubChem | |
URL | https://pubchem.ncbi.nlm.nih.gov | |
Description | Data deposited in or computed by PubChem | |
Molecular Weight |
320.0 g/mol | |
Details | Computed by PubChem 2.1 (PubChem release 2021.05.07) | |
Source | PubChem | |
URL | https://pubchem.ncbi.nlm.nih.gov | |
Description | Data deposited in or computed by PubChem | |
Retrosynthesis Analysis
AI-Powered Synthesis Planning: Our tool employs the Template_relevance Pistachio, Template_relevance Bkms_metabolic, Template_relevance Pistachio_ringbreaker, Template_relevance Reaxys, Template_relevance Reaxys_biocatalysis model, leveraging a vast database of chemical reactions to predict feasible synthetic routes.
One-Step Synthesis Focus: Specifically designed for one-step synthesis, it provides concise and direct routes for your target compounds, streamlining the synthesis process.
Accurate Predictions: Utilizing the extensive PISTACHIO, BKMS_METABOLIC, PISTACHIO_RINGBREAKER, REAXYS, REAXYS_BIOCATALYSIS database, our tool offers high-accuracy predictions, reflecting the latest in chemical research and data.
Strategy Settings
Precursor scoring | Relevance Heuristic |
---|---|
Min. plausibility | 0.01 |
Model | Template_relevance |
Template Set | Pistachio/Bkms_metabolic/Pistachio_ringbreaker/Reaxys/Reaxys_biocatalysis |
Top-N result to add to graph | 6 |
Feasible Synthetic Routes
Disclaimer and Information on In-Vitro Research Products
Please be aware that all articles and product information presented on BenchChem are intended solely for informational purposes. The products available for purchase on BenchChem are specifically designed for in-vitro studies, which are conducted outside of living organisms. In-vitro studies, derived from the Latin term "in glass," involve experiments performed in controlled laboratory settings using cells or tissues. It is important to note that these products are not categorized as medicines or drugs, and they have not received approval from the FDA for the prevention, treatment, or cure of any medical condition, ailment, or disease. We must emphasize that any form of bodily introduction of these products into humans or animals is strictly prohibited by law. It is essential to adhere to these guidelines to ensure compliance with legal and ethical standards in research and experimentation.