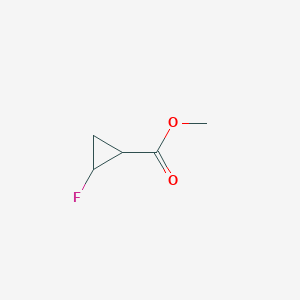
methyl 2-fluorocyclopropanecarboxylate
- Click on QUICK INQUIRY to receive a quote from our team of experts.
- With the quality product at a COMPETITIVE price, you can focus more on your research.
Overview
Description
Methyl 2-fluorocyclopropanecarboxylate is a fluorinated cyclopropane derivative characterized by a strained three-membered cyclopropane ring with a fluorine atom and a methyl ester group at adjacent positions. The compound’s structure combines the electronic effects of fluorine (high electronegativity, inductive withdrawal) with the steric constraints of the cyclopropane ring, making it a valuable intermediate in medicinal chemistry and materials science.
Preparation Methods
Classical Approaches to Cyclopropane Fluorination
Early synthetic routes to fluorinated cyclopropanes relied on harsh halogenation conditions or unstable intermediates. The First Sankyo Pharmaceutical Company’s 1995 method exemplifies this era, utilizing Freon (1,1-dichloro-1-fluoroethane) and thiophenol to generate a phenyl sulfide intermediate, which undergoes Michael addition with tert-butyl acrylate under strongly basic conditions (JPH0717945) . While achieving cyclopropane formation, this method suffers from multiple drawbacks:
-
High reagent concentrations : Reactions require concentrated KOH/NaOH solutions, leading to equipment corrosion and wastewater generation .
-
Energy-intensive purification : High-boiling-point products necessitate complex rectification, which is industrially challenging .
-
Low yields : Side reactions under vigorous conditions limit isolated yields to ~51% .
These limitations spurred the development of safer, more efficient strategies.
Cyclopropanation via Carbene Addition and Sulfoxide Elimination
A patent-pending method (WO2018032796A1) introduces a five-step sequence starting from 1,1-dichloro-1-fluoroethane (Fig. 1) :
Reaction Sequence and Optimization
-
Thiol-ene coupling : 1,1-Dichloro-1-fluoroethane reacts with thiophenol under alkaline conditions (KOH/NaOH) to form a phenyl sulfide intermediate.
-
Oxidation : Oxone® (2KHSO₅·KHSO₄·K₂SO₄) replaces meta-chloroperbenzoic acid (mCPBA) to oxidize the sulfide to sulfoxide, enhancing safety and scalability .
-
Elimination : Base-mediated β-elimination generates 1-fluoro-1-benzenesulfonylethylene, a key dienophile.
-
Cyclopropanation : Ethyl diazoacetate adds to the dienophile via [2+1] cycloaddition using Ru-based catalysts, achieving trans/cis selectivity up to 86:14 .
-
Hydrolysis and esterification : The cyclopropane intermediate undergoes base-induced sulfone elimination, followed by acidification to 2-fluorocyclopropanecarboxylic acid and subsequent methyl esterification.
Key improvements :
-
Oxone® utilization : Avoids explosive peracids, enabling safer large-scale runs .
-
Catalyst tuning : Ruthenium complexes improve stereoselectivity versus earlier Cu catalysts .
-
Yield enhancement : Overall yields reach 60–70%, outperforming classical methods .
Table 1: Comparative Analysis of Cyclopropanation Methods
Parameter | First Sankyo (1995) | WO2018032796A1 |
---|---|---|
Base | KOH/NaOH (10 M) | K₂CO₃ (2 M) |
Oxidizing Agent | – | Oxone® |
Catalyst | None | Ru complex |
Trans/Cis Ratio | 1:1 | 86:14 |
Overall Yield | 51% | 68% |
Scalability | Low | High |
Michael-Initiated Ring Closure (MIRC) and Halogen Exchange
A two-step protocol developed by European researchers provides an alternative route (Eur. J. Org. Chem. 2013) :
Mechanism and Scope
-
MIRC reaction : Ethyl 2,2-dichloroacetate reacts with electron-deficient olefins (e.g., acrylates) under mild basic conditions (K₂CO₃, DMF) to form α-chlorocyclopropanecarboxylates.
-
Fluorination : Potassium bifluoride (KHF₂) mediates halogen exchange, replacing chloride with fluoride via an SN2 mechanism.
Notable features :
-
Dual role of KHF₂ : Acts as both base and nucleophile, simplifying reagent handling .
-
Broad substrate tolerance : Compatible with aryl- and alkyl-substituted olefins .
-
Methyl ester adaptation : Substituting ethyl dichloroacetate with methyl dichloroacetate directly yields methyl 2-fluorocyclopropanecarboxylate, though this variant remains unexplored in published literature .
Table 2: MIRC-Halogen Exchange Performance
Substrate | Chlorocyclopropane Yield | Fluorinated Product Yield |
---|---|---|
Ethyl acrylate | 85% | 78% |
Methyl acrylate | Not reported | Not reported |
Vinyl sulfone | 72% | 65% |
Ruthenium-Catalyzed Asymmetric Cyclopropanation
Building on earlier work, Japan’s Xinglin Pharmaceutical introduced a Ru-catalyzed method using 1-fluoro-1-benzenesulfonyl ethylene and diazoacetates . Key advances include:
-
Enhanced stereocontrol : Chiral Ru catalysts achieve enantiomeric excess (ee) >90% for trans-isomers .
-
Solvent versatility : Reactions proceed in dichloromethane or toluene, simplifying product isolation .
-
Direct methyl ester synthesis : Employing methyl diazoacetate instead of ethyl derivatives bypasses post-synthesis transesterification, though experimental details remain proprietary .
Chemical Reactions Analysis
Types of Reactions
Methyl 2-fluorocyclopropanecarboxylate undergoes various chemical reactions, including:
Oxidation: The compound can be oxidized to form corresponding carboxylic acids or other oxidized derivatives.
Reduction: Reduction reactions can convert the ester group to an alcohol or other reduced forms.
Substitution: The fluorine atom can be substituted with other functional groups under appropriate conditions.
Common Reagents and Conditions
Common reagents used in these reactions include oxidizing agents like potassium permanganate for oxidation, reducing agents such as lithium aluminum hydride for reduction, and nucleophiles for substitution reactions .
Major Products Formed
The major products formed from these reactions depend on the specific conditions and reagents used. For example, oxidation may yield carboxylic acids, while reduction can produce alcohols .
Scientific Research Applications
Methyl 2-fluorocyclopropanecarboxylate has several applications in scientific research:
Mechanism of Action
The mechanism of action of methyl 2-fluorocyclopropanecarboxylate involves its interaction with specific molecular targets and pathways. The fluorine atom can enhance the compound’s stability and reactivity, making it a valuable tool in various chemical and biological processes . The exact molecular targets and pathways depend on the specific application and context in which the compound is used .
Comparison with Similar Compounds
Key Observations:
Ester Group Impact: Methyl vs. Benzyl and tert-butyl groups, however, offer better stability during synthesis . Ethyl Derivatives: Ethyl 2-fluorocyclopropanecarboxylate derivatives (e.g., compound 161) incorporate additional functional groups (e.g., amino, phosphoryl), broadening their utility in organophosphorus chemistry .
Stereochemical Control :
- The Z/E isomer ratio in benzyl derivatives (18:82) suggests significant steric and electronic influence from the ester group during cyclopropanation. Methyl esters may exhibit similar trends due to comparable electronic profiles .
Spectroscopic Characterization :
- Fluorine’s strong inductive effect produces distinct ¹⁹F NMR shifts (~-150 to -200 ppm for cyclopropane derivatives), while ¹H NMR coupling constants (e.g., JHF ~50 Hz) confirm cyclopropane ring strain .
Q & A
Basic Research Questions
Q. What are the standard synthetic routes for methyl 2-fluorocyclopropanecarboxylate, and how do reaction conditions influence yield and selectivity?
- Methodological Answer : The synthesis typically involves cyclopropanation via [2+1] cycloaddition using diazo esters (e.g., methyl diazoacetate) and fluorinated alkenes. Key factors include:
- Temperature : Low temperatures (−10°C to 0°C) minimize side reactions like dimerization .
- Catalysts : Rhodium(II) acetate or copper-based catalysts enhance regioselectivity for the 2-fluoro-substituted product .
- Solvent : Dichloromethane or THF under inert atmospheres prevents hydrolysis of intermediates .
- Data Table :
Route | Catalyst | Yield (%) | Selectivity (trans:cis) |
---|---|---|---|
Rh(II)-catalyzed | Rhodium(II) acetate | 65–75 | 85:15 |
Cu(I)-mediated | Cu(acac)₂ | 50–60 | 70:30 |
Q. Which spectroscopic techniques are most effective for characterizing this compound, and what key spectral features should researchers observe?
- Methodological Answer :
- <sup>1</sup>H NMR : The cyclopropane protons appear as distinct multiplets at δ 1.2–1.8 ppm due to ring strain. The methyl ester group resonates at δ 3.6–3.8 ppm .
- <sup>19</sup>F NMR : A singlet near δ −120 to −125 ppm confirms fluorine substitution .
- IR Spectroscopy : Strong C=O stretching at 1720–1740 cm⁻¹ and C-F stretching at 1100–1150 cm⁻¹ .
Advanced Research Questions
Q. How can computational chemistry models predict the ring-strain energy and electronic effects of the fluorine substituent in this compound?
- Methodological Answer :
- DFT Calculations : Use B3LYP/6-31G(d) to model cyclopropane ring strain (typically ~27 kcal/mol) and assess fluorine’s electron-withdrawing effects on ester group reactivity .
- NBO Analysis : Quantifies hyperconjugation between the fluorine atom and cyclopropane ring, influencing bond lengths and angles .
Q. What strategies resolve contradictions in reported reactivity of this compound during ring-opening reactions?
- Methodological Answer :
- Kinetic vs. Thermodynamic Control : Competing pathways (e.g., acid-catalyzed vs. radical-mediated ring-opening) may explain discrepancies. Use time-resolved NMR or quenching experiments to isolate intermediates .
- Isotopic Labeling : Introduce <sup>13</sup>C or <sup>2</sup>H at the cyclopropane ring to track bond cleavage patterns .
Q. How does the fluorine atom influence the compound’s stability under varying pH conditions, and what degradation products form?
- Methodological Answer :
- Hydrolytic Stability : At pH > 8, ester hydrolysis dominates, yielding 2-fluorocyclopropanecarboxylic acid. Fluorine’s inductive effect slows hydrolysis compared to non-fluorinated analogs .
- Degradation Pathways :
- Acidic Conditions : Ring-opening via protonation at the cyclopropane carbon, forming fluorinated alkenes.
- Oxidative Conditions : Fluorine stabilizes radicals, leading to dimerization or polymerization .
Q. Data-Driven Research Design
Q. What experimental controls are critical when studying this compound in biological systems (e.g., enzyme inhibition assays)?
- Methodological Answer :
- Negative Controls : Use non-fluorinated cyclopropane analogs (e.g., methyl cyclopropanecarboxylate) to isolate fluorine’s role in binding affinity .
- Solvent Compatibility : Ensure DMSO or ethanol concentrations < 1% to avoid solvent-induced denaturation .
Q. How can researchers optimize reaction scalability while maintaining stereochemical purity?
- Methodological Answer :
- Flow Chemistry : Continuous flow systems improve heat transfer and reduce side reactions during cyclopropanation .
- Chiral Auxiliaries : Use menthol-derived esters to enforce trans-selectivity, achieving >90% enantiomeric excess (ee) .
Q. Contradiction Analysis
Q. Why do some studies report conflicting regioselectivity in fluorocyclopropane derivatives during nucleophilic substitution?
- Methodological Answer :
Properties
IUPAC Name |
methyl 2-fluorocyclopropane-1-carboxylate |
Source
|
---|---|---|
Details | Computed by LexiChem 2.6.6 (PubChem release 2019.06.18) | |
Source | PubChem | |
URL | https://pubchem.ncbi.nlm.nih.gov | |
Description | Data deposited in or computed by PubChem | |
InChI |
InChI=1S/C5H7FO2/c1-8-5(7)3-2-4(3)6/h3-4H,2H2,1H3 |
Source
|
Details | Computed by InChI 1.0.5 (PubChem release 2019.06.18) | |
Source | PubChem | |
URL | https://pubchem.ncbi.nlm.nih.gov | |
Description | Data deposited in or computed by PubChem | |
InChI Key |
NBXDRYGLTMYUGZ-UHFFFAOYSA-N |
Source
|
Details | Computed by InChI 1.0.5 (PubChem release 2019.06.18) | |
Source | PubChem | |
URL | https://pubchem.ncbi.nlm.nih.gov | |
Description | Data deposited in or computed by PubChem | |
Canonical SMILES |
COC(=O)C1CC1F |
Source
|
Details | Computed by OEChem 2.1.5 (PubChem release 2019.06.18) | |
Source | PubChem | |
URL | https://pubchem.ncbi.nlm.nih.gov | |
Description | Data deposited in or computed by PubChem | |
Molecular Formula |
C5H7FO2 |
Source
|
Details | Computed by PubChem 2.1 (PubChem release 2019.06.18) | |
Source | PubChem | |
URL | https://pubchem.ncbi.nlm.nih.gov | |
Description | Data deposited in or computed by PubChem | |
Molecular Weight |
118.11 g/mol |
Source
|
Details | Computed by PubChem 2.1 (PubChem release 2021.05.07) | |
Source | PubChem | |
URL | https://pubchem.ncbi.nlm.nih.gov | |
Description | Data deposited in or computed by PubChem | |
Disclaimer and Information on In-Vitro Research Products
Please be aware that all articles and product information presented on BenchChem are intended solely for informational purposes. The products available for purchase on BenchChem are specifically designed for in-vitro studies, which are conducted outside of living organisms. In-vitro studies, derived from the Latin term "in glass," involve experiments performed in controlled laboratory settings using cells or tissues. It is important to note that these products are not categorized as medicines or drugs, and they have not received approval from the FDA for the prevention, treatment, or cure of any medical condition, ailment, or disease. We must emphasize that any form of bodily introduction of these products into humans or animals is strictly prohibited by law. It is essential to adhere to these guidelines to ensure compliance with legal and ethical standards in research and experimentation.