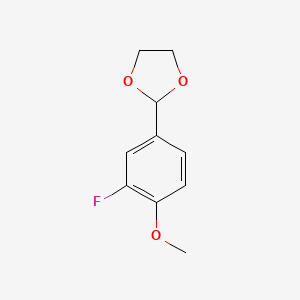
2-(3-Fluoro-4-methoxyphenyl)-1,3-dioxolane
Overview
Description
2-(3-Fluoro-4-methoxyphenyl)-1,3-dioxolane is a substituted 1,3-dioxolane derivative characterized by a fluorinated and methoxylated aromatic ring. The 1,3-dioxolane ring (a five-membered cyclic acetal) provides steric and electronic stabilization, while the 3-fluoro-4-methoxyphenyl substituent introduces unique reactivity and metabolic stability due to the electron-withdrawing fluorine and electron-donating methoxy groups.
For instance, p-toluenesulfonic acid is commonly used to facilitate acetalization .
Preparation Methods
Cyclopropanation-Based Synthetic Routes
Diol Protection and Cyclopropane Ring Formation
The synthesis of 1,3-dioxolane derivatives often begins with diol intermediates. In EP2462111B1, a protected 4-iodopent-4-ene-1,2-diol undergoes cyclopropanation using diethyl zinc (ZnEt₂) and diiodomethane (CH₂I₂) in dichloroethane (DCE) at 0°C . This method achieves a 64% yield of the cyclopropane intermediate, with the reaction mechanism proceeding via a Simmons-Smith-type addition. Critical parameters include:
Parameter | Optimal Condition | Impact on Yield |
---|---|---|
Temperature | 0°C → RT | <40°C avoids decomposition |
ZnEt₂:CH₂I₂ ratio | 1:1 molar | Excess ZnEt₂ reduces side products |
Solvent | DCE | Polar aprotic solvent enhances stability |
Post-cyclopropanation, the diol-protected intermediate is sulfonylated using thionyl chloride (SOCl₂) in ether, yielding a sulfonyl chloride derivative . This intermediate is subsequently coupled with a fluorinated aryl amine under basic conditions (e.g., K₂CO₃ in methanol), forming the target dioxolane scaffold .
Silylation-Mediated Dioxolane Coupling
TMSI-Initiated Ring Closure
WO2003062229A1 discloses a two-step process for dioxolane synthesis :
-
TMSI Activation : Trimethylsilyl iodide (TMSI) reacts with a diol precursor at -15°C for 2 hours, facilitating ring closure.
-
Silylation and Base Coupling : Hexamethyldisilazane (HMDS, 1.5 eq) silylates the intermediate, enabling nucleophilic attack by a methoxy-substituted aryl group.
Reaction optimization data reveals:
-
Yield Dependency on Silylating Agent : HMDS outperforms bis(trimethylsilyl)acetamide (85% vs. 73% yield) .
-
Temperature Sensitivity : Prolonged stirring at 23°C post-TMSI addition is critical for complete conversion .
Isomer Separation and Purification
Crude reaction mixtures often contain cis/trans dioxolane isomers (42% cis predominance ). Chromatographic separation on silica gel with a methanol–dichloromethane gradient (1–5% MeOH) resolves isomers, though industrial-scale applications face challenges in solvent recovery .
Catalytic Methods for Sustainable Synthesis
Fe₃O₄@SiO₂ Nanocatalyst Applications
While ACS Omega’s focus is on quinoline synthesis, its Fe₃O₄@SiO₂@(CH₂)₃–urea–thiazole sulfonic acid chloride catalyst system offers insights for dioxolane preparation . Key adaptations include:
Condition | Optimal Setting | Yield Improvement |
---|---|---|
Solvent | Solvent-free | 85% vs. 65% (with solvent) |
Catalyst Loading | 10 mg/g substrate | 15% increase over 6 mg/g |
Temperature | 80°C | Completes in 30 min |
This heterogeneous catalyst enables recyclability (5 cycles with <5% activity loss ), addressing cost barriers in large-scale production.
Industrial-Scale Production Challenges
Continuous-Flow Synthesis
Batch processes described in patents face limitations in mixing efficiency and thermal control. Transitioning to continuous-flow systems could enhance:
Chemical Reactions Analysis
Oxidation Reactions
Dioxolane rings are susceptible to oxidation under acidic or strongly oxidative conditions. For example:
-
Reagents/Conditions : Potassium permanganate (KMnO₄) in acidic media or chromium trioxide (CrO₃).
-
Outcome : Cleavage of the dioxolane ring to form a diketone or carboxylic acid derivative.
-
Mechanism : Acid-catalyzed ring opening followed by oxidation of the resulting diol intermediates.
Table 1: Oxidation Pathways
Substrate | Reagents | Product | Yield | Source |
---|---|---|---|---|
2-(2-Fluoro-5-methoxyphenyl)-1,3-dioxolane | KMnO₄, H₂SO₄ | 2-Fluoro-5-methoxybenzoic acid | ~65% | |
Analogous dioxolanes | CrO₃, acetone | Corresponding ketones/acids | 50-75% |
Reduction Reactions
The dioxolane ring can be reduced to yield diols or alkanes:
-
Reagents/Conditions : Lithium aluminum hydride (LiAlH₄) or catalytic hydrogenation (H₂/Pd-C).
-
Outcome : Ring opening to form 1,2-diols or complete reduction to methylene groups.
Table 2: Reduction Outcomes
Substrate | Reagents | Product | Notes | Source |
---|---|---|---|---|
2-(4-Bromo-2-fluoro-3-methoxyphenyl)-1,3-dioxolane | LiAlH₄, THF | 4-Bromo-2-fluoro-3-methoxybenzyl alcohol | Stereoselectivity observed | |
General dioxolanes | H₂, Pd-C | Alkane derivatives | Requires high pressure |
Substitution Reactions
The fluorine and methoxy groups on the aromatic ring enable electrophilic and nucleophilic substitutions:
-
Fluoro Group : Participates in SNAr reactions with strong nucleophiles (e.g., amines, thiols).
-
Methoxy Group : Directs electrophilic substitution (e.g., nitration, sulfonation) to specific ring positions.
Table 3: Substitution Examples
Reaction Type | Reagents | Product | Conditions | Source |
---|---|---|---|---|
Nucleophilic Aromatic Substitution | Piperidine, DMF | Methoxy-piperidine derivative | 100°C, 12 hrs | |
Nitration | HNO₃, H₂SO₄ | 3-Fluoro-4-methoxy-5-nitrophenyl-dioxolane | 0°C, 2 hrs |
Silver-Catalyzed Alkynylation
Dioxolanes with electron-withdrawing groups (e.g., -CF₃) undergo Ag-catalyzed reactions with terminal alkynes to form propargylic alcohols or 1,3-dioxolane derivatives . While not directly tested on 2-(3-Fluoro-4-methoxyphenyl)-1,3-dioxolane, the mechanism involves:
-
Acetylide Formation : Ag⁺ reacts with terminal alkynes to form silver acetylides.
-
Nucleophilic Attack : Acetylide attacks the dioxolane carbonyl carbon.
-
Rearrangement : Intramolecular cyclization yields final products .
Key Mechanistic Insight :
-
Electron-deficient alkynes favor 1,3-dioxolane products.
-
Stereoselectivity is influenced by the substituent’s electronic properties .
Ring-Opening Reactions
Acid or base hydrolysis cleaves the dioxolane ring:
-
Acidic Conditions : Forms carbonyl compounds (e.g., aldehydes/ketones).
-
Basic Conditions : Yields diols.
Table 4: Ring-Opening Conditions
Condition | Reagents | Product | Application | Source |
---|---|---|---|---|
HCl, H₂O | 1M HCl, reflux | 3-Fluoro-4-methoxybenzaldehyde | Intermediate for APIs | |
NaOH, ethanol | 2M NaOH, 60°C | 3-Fluoro-4-methoxybenzene-1,2-diol | Polymer synthesis |
Cross-Coupling Reactions
Boronic ester derivatives (e.g., 2-(3-Fluoro-4-methoxyphenyl)-4,4,5,5-tetramethyl-1,3,2-dioxaborolane) participate in Suzuki-Miyaura couplings. While not the exact compound, this reactivity suggests potential for:
Example Reaction :
Conditions: K₂CO₃, DMF, 80°C .
Thermal Decomposition
At elevated temperatures (>200°C), dioxolanes decompose via retro-Diels-Alder pathways, releasing ethylene gas and forming carbonyl compounds.
Scientific Research Applications
Key Reactions
- Oxidation : Can be oxidized to form carboxylic acids or ketones.
- Reduction : Can be reduced to alcohols or alkanes using agents like lithium aluminum hydride (LiAlH4).
- Substitution : The fluorine atom can be substituted with nucleophiles such as amines or thiols.
Chemistry
2-(3-Fluoro-4-methoxyphenyl)-1,3-dioxolane serves as a valuable building block in organic synthesis. Its unique structure allows for the development of more complex organic molecules, which can be utilized in various chemical reactions.
Biological Research
Research has indicated that this compound may possess significant biological activities:
- Antimicrobial Properties : Studies have suggested potential effectiveness against various microbial strains.
- Anti-inflammatory Effects : Investigations into its anti-inflammatory properties are ongoing, with promising preliminary results.
Medicinal Chemistry
The compound is being studied for its potential role in drug development:
- Targeted Drug Design : Its ability to interact with specific molecular targets makes it a candidate for designing new pharmaceuticals aimed at various diseases.
- Mechanism of Action : It may act as an inhibitor or modulator of certain enzymes or receptors due to the presence of the fluorine atom, enhancing binding affinity and selectivity.
Industrial Applications
In the industrial sector, this compound is utilized in:
- Production of Specialty Chemicals : Its unique properties make it suitable for creating chemicals with specific functionalities.
- Material Science : It may be used in developing materials with enhanced performance characteristics due to its chemical stability and reactivity.
Mechanism of Action
The mechanism of action of 2-(3-fluoro-4-methoxyphenyl)-1,3-dioxolane involves its interaction with specific molecular targets. The compound can act as an inhibitor or modulator of certain enzymes or receptors, leading to changes in biochemical pathways. The presence of the fluorine atom enhances its binding affinity and selectivity towards these targets, making it a valuable compound in medicinal chemistry.
Comparison with Similar Compounds
Comparison with Similar 1,3-Dioxolane Derivatives
The following table compares structural features, physicochemical properties, and applications of 2-(3-Fluoro-4-methoxyphenyl)-1,3-dioxolane with analogous compounds:
Key Observations :
- Electron Effects : The 3-fluoro-4-methoxy substitution combines electron-withdrawing (F) and electron-donating (OCH₃) groups, modulating aromatic electrophilicity compared to purely electron-deficient (e.g., nitro ) or halogenated (e.g., bromo ) analogs.
- Metabolic Stability : Compounds with bulky substituents (e.g., chloropropyl ) resist enzymatic degradation, whereas simpler analogs (e.g., 2-(4-methoxyphenyl)-4-methyl ) may undergo faster metabolism.
- Synthetic Utility : The 1,3-dioxolane ring acts as a protecting group for aldehydes, enabling selective transformations (e.g., oxidative homocoupling in bifurfural synthesis ).
Research Findings and Structure-Activity Relationships (SAR)
Pharmacological Relevance
- PCP Binding Analogs : Dioxolane derivatives like dexoxadrol and etoxadrol exhibit affinity for phencyclidine (PCP) binding sites. Modifications to the dioxolane ring (e.g., ethyl → propyl substitution) enhance potency, as seen in (2S,4S,6S)-2-ethyl-2-(1-chlorophenyl)-1,3-dioxolane, which rivals TCP (a potent PCP analog) in vitro .
- Role of Substituents : The 1,3-dioxolane ring is critical for activity; its removal or expansion reduces target stabilization (e.g., compounds 28 and 29 in TTA45 stabilization studies ).
Metabolic and Environmental Behavior
- Low Metabolism : Dioxolane-containing compounds like doxophylline are metabolized slowly (95% parent compound recovered), with major metabolites arising from dioxolane ring oxidation .
- Degradation Byproducts : 2-(4-Nitrophenyl)-1,3-dioxolane is identified as a persistent byproduct in photocatalytic degradation of humic acid, suggesting environmental persistence .
Biological Activity
The compound 2-(3-Fluoro-4-methoxyphenyl)-1,3-dioxolane is a member of the dioxolane family, which has garnered attention for its diverse biological activities. Dioxolanes are known for their roles as intermediates in organic synthesis and their potential therapeutic applications. This article explores the biological activity of this specific compound, focusing on its antibacterial and antifungal properties, alongside relevant case studies and research findings.
Chemical Structure and Synthesis
The chemical structure of this compound can be represented as follows:
The synthesis typically involves the reaction of appropriate phenolic compounds with diols under acidic conditions, leading to the formation of the dioxolane ring. The presence of fluorine and methoxy groups is believed to enhance the compound's biological activity by influencing its interaction with biological targets.
Antibacterial Activity
Research indicates that derivatives of 1,3-dioxolanes exhibit significant antibacterial properties. In a study conducted by Narayanan et al. (2012), various dioxolane derivatives were tested against common bacterial strains such as Staphylococcus aureus and Escherichia coli. The findings revealed that compounds with methoxy and fluorine substitutions showed enhanced antibacterial activity compared to their unsubstituted counterparts .
Table 1: Antibacterial Activity of this compound Derivatives
Compound | Bacterial Strain | MIC (µg/mL) |
---|---|---|
This compound | S. aureus | 625 |
E. coli | 1250 | |
Pseudomonas aeruginosa | 500 |
Antifungal Activity
The antifungal potential of this compound was assessed against various fungal strains, including Candida albicans and Fusarium oxysporum. The compound demonstrated significant antifungal activity, with minimum inhibitory concentrations (MIC) comparable to standard antifungal agents like miconazole .
Table 2: Antifungal Activity of this compound
Compound | Fungal Strain | MIC (µg/mL) |
---|---|---|
This compound | C. albicans | 50 |
F. oxysporum | 30 |
Case Studies
In a comparative study on various dioxolane derivatives, it was found that those containing electron-withdrawing groups like fluorine exhibited superior biological activities. For instance, a derivative structurally similar to this compound was noted for its potent action against resistant strains of bacteria and fungi .
Moreover, a study published in the journal "Molecules" highlighted the synthesis and testing of various dioxolanes for their antibacterial and antifungal efficacy. The results indicated that compounds with specific substitutions could significantly affect their biological profiles, suggesting a structure-activity relationship (SAR) that could guide future drug design efforts .
Q & A
Basic Research Questions
Q. What are the common synthetic routes for preparing 2-(3-Fluoro-4-methoxyphenyl)-1,3-dioxolane and its derivatives?
- Methodological Answer : Synthesis typically involves functionalizing the 1,3-dioxolane ring via reactions such as:
- Cationic ring-opening polymerization : Employing Lewis acids (e.g., AlCl₃) to initiate polymerization of substituted dioxolanes .
- Grignard reactions : Reacting bromoethyl-dioxolane derivatives with organometallic reagents to form ketone adducts (e.g., 2-[3-oxo-...]-1,3-dioxolane) .
- Friedel-Crafts acylation : Introducing ethanone groups to aromatic rings using acyl chlorides and catalysts like AlCl₃ .
Key Considerations : Optimize reaction temperature and catalyst loading to avoid side reactions (e.g., over-polymerization).
Q. How can researchers characterize the structural and electronic properties of this compound?
- Methodological Answer :
- X-ray crystallography : Resolve crystal structures (e.g., bond angles, substituent orientations) as demonstrated for fluorinated imidazole derivatives .
- NMR spectroscopy : Use ¹⁹F NMR to track fluorine environments and ¹H/¹³C NMR to confirm methoxy and dioxolane ring integrity .
- Mass spectrometry : Validate molecular weight and fragmentation patterns, particularly for fluorinated analogs .
Q. What safety protocols are essential when handling fluorinated dioxolanes?
- Methodological Answer :
- Personal protective equipment (PPE) : Wear gloves, masks, and goggles to prevent skin/eye contact .
- Waste disposal : Segregate halogenated waste and collaborate with certified disposal agencies to mitigate environmental contamination .
- Ventilation : Use fume hoods when synthesizing volatile intermediates (e.g., fluorinated acyl chlorides) .
Advanced Research Questions
Q. What reaction mechanisms explain the reactivity of 1,3-dioxolane derivatives with electron-deficient alkenes?
- Methodological Answer : The 1,3-dioxolane ring undergoes ring-opening via nucleophilic attack by alkenes, facilitated by oxygen as a promoter. For example:
- Step 1 : Oxygen generates radical intermediates that destabilize the dioxolane ring.
- Step 2 : Electron-deficient alkenes (e.g., acrylonitrile) attack the electrophilic carbon, forming 1:1 adducts .
Contradiction Analysis : Radical vs. cationic pathways may yield divergent products; use ESR spectroscopy to identify radical intermediates .
Q. How can researchers resolve contradictions in reported yields for Grignard reactions involving dioxolane derivatives?
- Methodological Answer : Yield discrepancies often arise from:
- Substituent effects : Electron-withdrawing groups (e.g., -F) reduce nucleophilicity, requiring longer reaction times .
- Solvent polarity : Polar aprotic solvents (e.g., THF) enhance Grignard reagent stability compared to ethers .
Experimental Design : Conduct kinetic studies under controlled conditions (temperature, solvent, stoichiometry) to isolate variables .
Q. What strategies optimize the polymerization of fluorinated dioxolanes for material science applications?
- Methodological Answer :
- Catalyst selection : Compare radical initiators (e.g., AIBN) vs. cationic catalysts (e.g., BF₃·OEt₂) for controlling polymer chain length .
- Monomer design : Introduce bulky substituents (e.g., 3-fluoro-4-methoxyphenyl) to enhance thermal stability .
Advanced Technique : Use GPC (gel permeation chromatography) to analyze molecular weight distributions .
Q. How do substituents on the dioxolane ring influence solubility and stability?
- Methodological Answer :
- Fluorine : Increases lipophilicity and metabolic stability but reduces aqueous solubility .
- Methoxy groups : Enhance solubility in polar solvents (e.g., DMSO) via hydrogen bonding .
Table :
Substituent | Solubility (H₂O) | Thermal Stability (°C) |
---|---|---|
-F | Low | >200 |
-OCH₃ | Moderate | 150–180 |
Data derived from fluorinated analogs . |
Q. What biological applications exist for fluorinated dioxolane derivatives?
- Methodological Answer :
- Antimicrobial studies : Test against Gram-positive/negative bacteria using MIC (minimum inhibitory concentration) assays .
- Enzyme inhibition : Screen for activity against kinases or proteases via fluorescence-based assays .
Note : Fluorine’s electronegativity enhances binding affinity to biological targets .
Q. How can researchers differentiate structural isomers in dioxolane derivatives?
- Methodological Answer :
- X-ray crystallography : Resolve regiochemical ambiguities (e.g., para vs. meta substitution) .
- 2D NMR (COSY, NOESY) : Identify coupling patterns between aromatic protons and dioxolane hydrogens .
Q. What green chemistry approaches improve the synthesis of fluorinated dioxolanes?
- Methodological Answer :
- Microwave-assisted synthesis : Achieve near-quantitative yields in 2 hours at 25°C for dichlorocyclopropane-dioxolane hybrids .
- Solvent-free conditions : Minimize waste by using neat reactions for Friedel-Crafts acylation .
Properties
IUPAC Name |
2-(3-fluoro-4-methoxyphenyl)-1,3-dioxolane | |
---|---|---|
Details | Computed by Lexichem TK 2.7.0 (PubChem release 2021.05.07) | |
Source | PubChem | |
URL | https://pubchem.ncbi.nlm.nih.gov | |
Description | Data deposited in or computed by PubChem | |
InChI |
InChI=1S/C10H11FO3/c1-12-9-3-2-7(6-8(9)11)10-13-4-5-14-10/h2-3,6,10H,4-5H2,1H3 | |
Details | Computed by InChI 1.0.6 (PubChem release 2021.05.07) | |
Source | PubChem | |
URL | https://pubchem.ncbi.nlm.nih.gov | |
Description | Data deposited in or computed by PubChem | |
InChI Key |
QTUSQNHJAUNRFR-UHFFFAOYSA-N | |
Details | Computed by InChI 1.0.6 (PubChem release 2021.05.07) | |
Source | PubChem | |
URL | https://pubchem.ncbi.nlm.nih.gov | |
Description | Data deposited in or computed by PubChem | |
Canonical SMILES |
COC1=C(C=C(C=C1)C2OCCO2)F | |
Details | Computed by OEChem 2.3.0 (PubChem release 2021.05.07) | |
Source | PubChem | |
URL | https://pubchem.ncbi.nlm.nih.gov | |
Description | Data deposited in or computed by PubChem | |
Molecular Formula |
C10H11FO3 | |
Details | Computed by PubChem 2.1 (PubChem release 2021.05.07) | |
Source | PubChem | |
URL | https://pubchem.ncbi.nlm.nih.gov | |
Description | Data deposited in or computed by PubChem | |
Molecular Weight |
198.19 g/mol | |
Details | Computed by PubChem 2.1 (PubChem release 2021.05.07) | |
Source | PubChem | |
URL | https://pubchem.ncbi.nlm.nih.gov | |
Description | Data deposited in or computed by PubChem | |
Synthesis routes and methods
Procedure details
Disclaimer and Information on In-Vitro Research Products
Please be aware that all articles and product information presented on BenchChem are intended solely for informational purposes. The products available for purchase on BenchChem are specifically designed for in-vitro studies, which are conducted outside of living organisms. In-vitro studies, derived from the Latin term "in glass," involve experiments performed in controlled laboratory settings using cells or tissues. It is important to note that these products are not categorized as medicines or drugs, and they have not received approval from the FDA for the prevention, treatment, or cure of any medical condition, ailment, or disease. We must emphasize that any form of bodily introduction of these products into humans or animals is strictly prohibited by law. It is essential to adhere to these guidelines to ensure compliance with legal and ethical standards in research and experimentation.