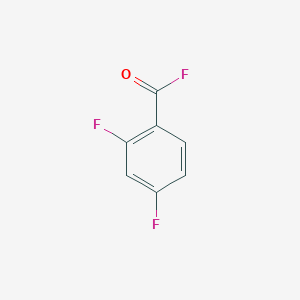
2,4-Difluorobenzoyl fluoride, 99%
- Click on QUICK INQUIRY to receive a quote from our team of experts.
- With the quality product at a COMPETITIVE price, you can focus more on your research.
Overview
Description
2,4-Difluorobenzoyl fluoride (also known as 2,4-Difluorobenzoyl chloride, 2,4-Difluorobenzene-1-sulfonyl chloride, and 2,4-Difluorobenzoyl sulfonyl chloride) is a fluorinated organic compound with the chemical formula C7H3F2O2S. It is a colorless and odorless liquid that is used in the synthesis of various organic and inorganic compounds. It is also used in pharmaceutical and agricultural applications.
Mechanism of Action
2,4-Difluorobenzoyl fluoride is an electrophilic fluorinating agent. It reacts with nucleophilic species to form a fluorinated product. The reaction is reversible, and the product can be further fluorinated or de-fluorinated. The reaction is catalyzed by Lewis acids, such as boron trifluoride.
Biochemical and Physiological Effects
2,4-Difluorobenzoyl fluoride is not known to have any significant biochemical or physiological effects on humans. However, it is important to note that it is a strong irritant to the skin and eyes, and should be handled with caution.
Advantages and Limitations for Lab Experiments
2,4-Difluorobenzoyl fluoride is a useful reagent for the synthesis of organic and inorganic compounds in the laboratory. It is relatively stable and can be stored at room temperature for extended periods of time. However, it is a strong irritant and must be handled with caution. It is also toxic and should not be inhaled or ingested.
Future Directions
There are several potential future directions for the use of 2,4-Difluorobenzoyl fluoride. It could be used in the synthesis of fluorinated polymers and surfactants. It could also be used in the synthesis of pharmaceuticals and agrochemicals. It could also be used in the synthesis of fluorinated drugs and other fluorinated compounds. Additionally, it could be used in the synthesis of fluorinated materials for medical applications. Finally, it could be used in the synthesis of fluorinated materials for industrial applications.
Synthesis Methods
The synthesis of 2,4-Difluorobenzoyl fluoride is carried out by the reaction of 2,4-difluorobenzaldehyde with phosphorus pentafluoride. The reaction is carried out in a dry, inert atmosphere at temperatures of -78 °C to -90 °C. The reaction is usually carried out in a sealed tube or flask to prevent the loss of volatile products.
Scientific Research Applications
2,4-Difluorobenzoyl fluoride is used in a variety of scientific research applications. It is used in the synthesis of organic and inorganic compounds, as well as in pharmaceutical and agricultural applications. It is also used in the synthesis of fluorinated drugs, as well as in the synthesis of other fluorinated compounds. It is also used in the synthesis of fluorinated polymers and in the synthesis of fluorinated surfactants.
properties
IUPAC Name |
2,4-difluorobenzoyl fluoride |
Source
|
---|---|---|
Details | Computed by LexiChem 2.6.6 (PubChem release 2019.06.18) | |
Source | PubChem | |
URL | https://pubchem.ncbi.nlm.nih.gov | |
Description | Data deposited in or computed by PubChem | |
InChI |
InChI=1S/C7H3F3O/c8-4-1-2-5(7(10)11)6(9)3-4/h1-3H |
Source
|
Details | Computed by InChI 1.0.5 (PubChem release 2019.06.18) | |
Source | PubChem | |
URL | https://pubchem.ncbi.nlm.nih.gov | |
Description | Data deposited in or computed by PubChem | |
InChI Key |
RTSJQGKALZWPTG-UHFFFAOYSA-N |
Source
|
Details | Computed by InChI 1.0.5 (PubChem release 2019.06.18) | |
Source | PubChem | |
URL | https://pubchem.ncbi.nlm.nih.gov | |
Description | Data deposited in or computed by PubChem | |
Canonical SMILES |
C1=CC(=C(C=C1F)F)C(=O)F |
Source
|
Details | Computed by OEChem 2.1.5 (PubChem release 2019.06.18) | |
Source | PubChem | |
URL | https://pubchem.ncbi.nlm.nih.gov | |
Description | Data deposited in or computed by PubChem | |
Molecular Formula |
C7H3F3O |
Source
|
Details | Computed by PubChem 2.1 (PubChem release 2019.06.18) | |
Source | PubChem | |
URL | https://pubchem.ncbi.nlm.nih.gov | |
Description | Data deposited in or computed by PubChem | |
DSSTOX Substance ID |
DTXSID90459725 |
Source
|
Record name | 2,4-difluorobenzoyl Fluoride | |
Source | EPA DSSTox | |
URL | https://comptox.epa.gov/dashboard/DTXSID90459725 | |
Description | DSSTox provides a high quality public chemistry resource for supporting improved predictive toxicology. | |
Molecular Weight |
160.09 g/mol |
Source
|
Details | Computed by PubChem 2.1 (PubChem release 2021.05.07) | |
Source | PubChem | |
URL | https://pubchem.ncbi.nlm.nih.gov | |
Description | Data deposited in or computed by PubChem | |
CAS RN |
67640-39-5 |
Source
|
Record name | 2,4-difluorobenzoyl Fluoride | |
Source | EPA DSSTox | |
URL | https://comptox.epa.gov/dashboard/DTXSID90459725 | |
Description | DSSTox provides a high quality public chemistry resource for supporting improved predictive toxicology. | |
Disclaimer and Information on In-Vitro Research Products
Please be aware that all articles and product information presented on BenchChem are intended solely for informational purposes. The products available for purchase on BenchChem are specifically designed for in-vitro studies, which are conducted outside of living organisms. In-vitro studies, derived from the Latin term "in glass," involve experiments performed in controlled laboratory settings using cells or tissues. It is important to note that these products are not categorized as medicines or drugs, and they have not received approval from the FDA for the prevention, treatment, or cure of any medical condition, ailment, or disease. We must emphasize that any form of bodily introduction of these products into humans or animals is strictly prohibited by law. It is essential to adhere to these guidelines to ensure compliance with legal and ethical standards in research and experimentation.