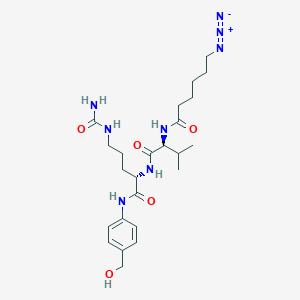
6-Azidohexanoyl-Val-Cit-PAB
- Click on QUICK INQUIRY to receive a quote from our team of experts.
- With the quality product at a COMPETITIVE price, you can focus more on your research.
Overview
Description
6-Azidohexanoyl-Val-Cit-PAB is a click chemistry reagent . It is used in the synthesis of vaccines . The chemical name for this compound is 6-azidohexanoyl-valyl-citrullyl-(4-aminobenzyl)-(4-nitrophenyl)-carbonate .
Molecular Structure Analysis
The molecular structure of 6-Azidohexanoyl-Val-Cit-PAB is composed of several functional groups . The molecular weight of this compound is 683.71, and its formula is C31H41N9O9 .Chemical Reactions Analysis
6-Azidohexanoyl-Val-Cit-PAB is used as a click chemistry reagent in the synthesis of vaccines . Click chemistry is a type of chemical reaction that joins small units together in a modular fashion, forming stable products.Physical And Chemical Properties Analysis
The physical and chemical properties of 6-Azidohexanoyl-Val-Cit-PAB include a molecular weight of 683.71 and a formula of C31H41N9O9 . Unfortunately, additional physical and chemical properties such as melting point, boiling point, and density were not found in the search results.Scientific Research Applications
Drug Delivery in Cancer Therapy
6-Azidohexanoyl-Val-Cit-PAB has been demonstrated to be effective in drug delivery systems targeting cancer cells. It offers straightforward drug attachment and effective cancer cell elimination, making it a promising agent for improved cancer therapy .
2. Click Chemistry Reagent for Vaccine Synthesis This compound serves as a click chemistry reagent, which is a method used to quickly and reliably join molecules together. It has been specifically mentioned for its use in vaccine synthesis, indicating its role in the development of new vaccines .
Pharmaceutical Testing and Reference Standards
6-Azidohexanoyl-Val-Cit-PAB is also utilized in pharmaceutical testing as a high-quality reference standard. This application ensures accurate results in pharmaceutical research, contributing to the development and quality control of new drugs .
Mechanism of Action
Target of Action
6-Azidohexanoyl-Val-Cit-PAB is primarily used in the synthesis of vaccines
Mode of Action
The compound 6-Azidohexanoyl-Val-Cit-PAB is a click chemistry reagent Click chemistry is a type of chemical synthesis characterized by its efficiency and versatility, often used in the creation of pharmaceuticals In the context of vaccine synthesis, this compound likely interacts with other molecules to form a complete vaccine structure
properties
IUPAC Name |
6-azido-N-[(2S)-1-[[(2S)-5-(carbamoylamino)-1-[4-(hydroxymethyl)anilino]-1-oxopentan-2-yl]amino]-3-methyl-1-oxobutan-2-yl]hexanamide |
Source
|
---|---|---|
Details | Computed by LexiChem 2.6.6 (PubChem release 2019.06.18) | |
Source | PubChem | |
URL | https://pubchem.ncbi.nlm.nih.gov | |
Description | Data deposited in or computed by PubChem | |
InChI |
InChI=1S/C24H38N8O5/c1-16(2)21(31-20(34)8-4-3-5-14-28-32-26)23(36)30-19(7-6-13-27-24(25)37)22(35)29-18-11-9-17(15-33)10-12-18/h9-12,16,19,21,33H,3-8,13-15H2,1-2H3,(H,29,35)(H,30,36)(H,31,34)(H3,25,27,37)/t19-,21-/m0/s1 |
Source
|
Details | Computed by InChI 1.0.5 (PubChem release 2019.06.18) | |
Source | PubChem | |
URL | https://pubchem.ncbi.nlm.nih.gov | |
Description | Data deposited in or computed by PubChem | |
InChI Key |
OVDFBASRMLDVQT-FPOVZHCZSA-N |
Source
|
Details | Computed by InChI 1.0.5 (PubChem release 2019.06.18) | |
Source | PubChem | |
URL | https://pubchem.ncbi.nlm.nih.gov | |
Description | Data deposited in or computed by PubChem | |
Canonical SMILES |
CC(C)C(C(=O)NC(CCCNC(=O)N)C(=O)NC1=CC=C(C=C1)CO)NC(=O)CCCCCN=[N+]=[N-] |
Source
|
Details | Computed by OEChem 2.1.5 (PubChem release 2019.06.18) | |
Source | PubChem | |
URL | https://pubchem.ncbi.nlm.nih.gov | |
Description | Data deposited in or computed by PubChem | |
Isomeric SMILES |
CC(C)[C@@H](C(=O)N[C@@H](CCCNC(=O)N)C(=O)NC1=CC=C(C=C1)CO)NC(=O)CCCCCN=[N+]=[N-] |
Source
|
Details | Computed by OEChem 2.1.5 (PubChem release 2019.06.18) | |
Source | PubChem | |
URL | https://pubchem.ncbi.nlm.nih.gov | |
Description | Data deposited in or computed by PubChem | |
Molecular Formula |
C24H38N8O5 |
Source
|
Details | Computed by PubChem 2.1 (PubChem release 2019.06.18) | |
Source | PubChem | |
URL | https://pubchem.ncbi.nlm.nih.gov | |
Description | Data deposited in or computed by PubChem | |
Molecular Weight |
518.6 g/mol |
Source
|
Details | Computed by PubChem 2.1 (PubChem release 2021.05.07) | |
Source | PubChem | |
URL | https://pubchem.ncbi.nlm.nih.gov | |
Description | Data deposited in or computed by PubChem | |
Product Name |
6-Azidohexanoyl-Val-Cit-PAB-PNP |
Disclaimer and Information on In-Vitro Research Products
Please be aware that all articles and product information presented on BenchChem are intended solely for informational purposes. The products available for purchase on BenchChem are specifically designed for in-vitro studies, which are conducted outside of living organisms. In-vitro studies, derived from the Latin term "in glass," involve experiments performed in controlled laboratory settings using cells or tissues. It is important to note that these products are not categorized as medicines or drugs, and they have not received approval from the FDA for the prevention, treatment, or cure of any medical condition, ailment, or disease. We must emphasize that any form of bodily introduction of these products into humans or animals is strictly prohibited by law. It is essential to adhere to these guidelines to ensure compliance with legal and ethical standards in research and experimentation.