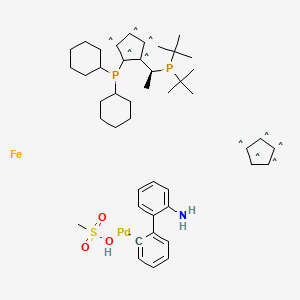
Bruno Palladacycle
- Click on QUICK INQUIRY to receive a quote from our team of experts.
- With the quality product at a COMPETITIVE price, you can focus more on your research.
Overview
Description
Bruno Palladacycle is a type of palladium complex that has gained significant attention in the field of catalysis, particularly in cross-coupling reactions. These compounds are characterized by the presence of a palladium atom bonded to a carbon atom within a cyclic structure. The unique properties of this compound make it a valuable catalyst in various chemical reactions, including carbon-carbon and carbon-nitrogen bond formation.
Mechanism of Action
Target of Action
Bruno Palladacycle, also known as “this compound, min. 98%”, primarily targets the C-C and C-N cross-coupling reactions . These reactions are crucial in the synthesis of complex organic compounds, particularly in the pharmaceutical industry .
Mode of Action
This compound interacts with its targets through a process known as palladium-catalyzed transformations . This compound serves as an intermediate in these transformations, facilitating the formation of new carbon-carbon (C-C) and carbon-nitrogen (C-N) bonds . The palladacycle’s unique electronic and steric properties help stabilize the catalytically active species and provide additional control over reaction selectivity .
Biochemical Pathways
The primary biochemical pathway affected by this compound is the Suzuki–Miyaura cross-coupling reaction . This reaction is a type of palladium-catalyzed cross-coupling method used to synthesize biaryl compounds, which are essential in various areas of chemistry . This compound also plays a role in the enantioselective β-C(sp3)-H arylation of carboxylic acids .
Result of Action
The action of this compound results in the formation of new C-C and C-N bonds, enabling the synthesis of complex organic compounds . This is particularly valuable in the pharmaceutical industry, where these reactions can lead to the creation of new drug molecules .
Action Environment
This suggests that the compound’s action, efficacy, and stability can be influenced by environmental factors such as the reaction medium .
Biochemical Analysis
Biochemical Properties
Bruno Palladacycle plays a significant role in biochemical reactions, particularly in catalytic applications. It has been found to be effective in facilitating Suzuki–Miyaura cross-couplings, a type of reaction that forms carbon-carbon bonds . The nature of these interactions involves the this compound acting as a pre-catalyst, transforming into a highly reactive, mono-ligated form of palladium(0), which then participates in the coupling reaction .
Molecular Mechanism
The molecular mechanism of this compound primarily involves its role as a catalyst in biochemical reactions. As a pre-catalyst, this compound undergoes a transformation to a highly reactive form of palladium(0), which then participates in the formation of carbon-carbon bonds in Suzuki–Miyaura cross-couplings . This process involves binding interactions with biomolecules, potentially leading to changes in gene expression .
Metabolic Pathways
Palladacycles are known to play a role in various biochemical reactions, suggesting that they may interact with certain enzymes or cofactors and potentially affect metabolic flux or metabolite levels .
Preparation Methods
Synthetic Routes and Reaction Conditions
Bruno Palladacycle can be synthesized through various methods. One common approach involves the reaction of palladium salts with ligands containing carbon-donor moieties. For example, the reaction of palladium acetate with a biphenyl ligand in the presence of a base can yield this compound. The reaction is typically carried out in an organic solvent such as dichloromethane at room temperature .
Industrial Production Methods
In an industrial setting, the production of this compound often involves the use of large-scale reactors and optimized reaction conditions to ensure high yield and purity. The process may include steps such as solvent extraction, crystallization, and purification through column chromatography .
Chemical Reactions Analysis
Types of Reactions
Bruno Palladacycle undergoes various types of chemical reactions, including:
Oxidation: this compound can be oxidized to form higher oxidation state palladium complexes.
Reduction: It can be reduced to generate palladium(0) species, which are highly reactive in catalytic cycles.
Substitution: Ligands in this compound can be substituted with other ligands, altering its catalytic properties.
Common Reagents and Conditions
Common reagents used in reactions involving this compound include bases such as sodium hydroxide, oxidizing agents like hydrogen peroxide, and reducing agents such as sodium borohydride. The reactions are typically carried out under mild conditions, often in aqueous or organic solvents .
Major Products
The major products formed from reactions involving this compound depend on the specific reaction conditions and reagents used. For example, in cross-coupling reactions, the major products are often biaryl compounds or amines .
Scientific Research Applications
Bruno Palladacycle has a wide range of applications in scientific research, including:
Comparison with Similar Compounds
Bruno Palladacycle can be compared with other similar compounds, such as:
N-Heterocyclic Carbene Palladacycles: These compounds also exhibit high catalytic activity but differ in their ligand structure and electronic properties.
Phosphine-Ligated Palladacycles: These palladacycles are known for their stability and ease of preparation but may have different reactivity profiles compared to this compound.
List of Similar Compounds
- N-Heterocyclic Carbene Palladacycles
- Phosphine-Ligated Palladacycles
- Symmetric Palladacycle Complexes
This compound stands out due to its unique ligand structure and the ability to function under mild reaction conditions, making it a versatile and valuable catalyst in various fields of research and industry.
Properties
InChI |
InChI=1S/C27H47P2.C12H10N.C5H5.CH4O3S.Fe.Pd/c1-21(29(26(2,3)4)27(5,6)7)24-19-14-20-25(24)28(22-15-10-8-11-16-22)23-17-12-9-13-18-23;13-12-9-5-4-8-11(12)10-6-2-1-3-7-10;1-2-4-5-3-1;1-5(2,3)4;;/h14,19-23H,8-13,15-18H2,1-7H3;1-6,8-9H,13H2;1-5H;1H3,(H,2,3,4);;/q;-1;;;;/t21-;;;;;/m0...../s1 |
Source
|
---|---|---|
Details | Computed by InChI 1.0.6 (PubChem release 2021.05.07) | |
Source | PubChem | |
URL | https://pubchem.ncbi.nlm.nih.gov | |
Description | Data deposited in or computed by PubChem | |
InChI Key |
INJPONIANSCTIE-DHOIFHORSA-N |
Source
|
Details | Computed by InChI 1.0.6 (PubChem release 2021.05.07) | |
Source | PubChem | |
URL | https://pubchem.ncbi.nlm.nih.gov | |
Description | Data deposited in or computed by PubChem | |
Canonical SMILES |
CC([C]1[CH][CH][CH][C]1P(C2CCCCC2)C3CCCCC3)P(C(C)(C)C)C(C)(C)C.CS(=O)(=O)O.C1=CC=C([C-]=C1)C2=CC=CC=C2N.[CH]1[CH][CH][CH][CH]1.[Fe].[Pd] |
Source
|
Details | Computed by OEChem 2.3.0 (PubChem release 2021.05.07) | |
Source | PubChem | |
URL | https://pubchem.ncbi.nlm.nih.gov | |
Description | Data deposited in or computed by PubChem | |
Isomeric SMILES |
C[C@@H]([C]1[CH][CH][CH][C]1P(C2CCCCC2)C3CCCCC3)P(C(C)(C)C)C(C)(C)C.CS(=O)(=O)O.C1=CC=C([C-]=C1)C2=CC=CC=C2N.[CH]1[CH][CH][CH][CH]1.[Fe].[Pd] |
Source
|
Details | Computed by OEChem 2.3.0 (PubChem release 2021.05.07) | |
Source | PubChem | |
URL | https://pubchem.ncbi.nlm.nih.gov | |
Description | Data deposited in or computed by PubChem | |
Molecular Formula |
C45H66FeNO3P2PdS- |
Source
|
Details | Computed by PubChem 2.1 (PubChem release 2021.05.07) | |
Source | PubChem | |
URL | https://pubchem.ncbi.nlm.nih.gov | |
Description | Data deposited in or computed by PubChem | |
Molecular Weight |
925.3 g/mol |
Source
|
Details | Computed by PubChem 2.1 (PubChem release 2021.05.07) | |
Source | PubChem | |
URL | https://pubchem.ncbi.nlm.nih.gov | |
Description | Data deposited in or computed by PubChem | |
Disclaimer and Information on In-Vitro Research Products
Please be aware that all articles and product information presented on BenchChem are intended solely for informational purposes. The products available for purchase on BenchChem are specifically designed for in-vitro studies, which are conducted outside of living organisms. In-vitro studies, derived from the Latin term "in glass," involve experiments performed in controlled laboratory settings using cells or tissues. It is important to note that these products are not categorized as medicines or drugs, and they have not received approval from the FDA for the prevention, treatment, or cure of any medical condition, ailment, or disease. We must emphasize that any form of bodily introduction of these products into humans or animals is strictly prohibited by law. It is essential to adhere to these guidelines to ensure compliance with legal and ethical standards in research and experimentation.