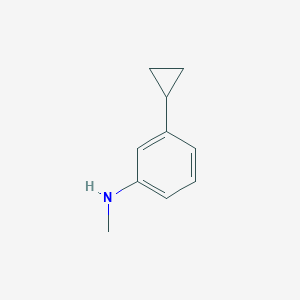
3-Cyclopropyl-N-methylaniline
- Click on QUICK INQUIRY to receive a quote from our team of experts.
- With the quality product at a COMPETITIVE price, you can focus more on your research.
Overview
Description
3-Cyclopropyl-N-methylaniline is an organic compound with the molecular formula C10H13N and a molecular weight of 147.22 g/mol. It is a derivative of aniline, where the hydrogen atom on the nitrogen is replaced by a methyl group, and a cyclopropyl group is attached to the benzene ring.
Mechanism of Action
Target of Action
The primary target of 3-Cyclopropyl-N-methylaniline is cytochrome P450 . Cytochrome P450 is a family of enzymes that play a key role in the metabolism of organic substances and the biosynthesis of important molecules.
Mode of Action
The compound interacts with its target, cytochrome P450, through a process called N-dealkylation . This reaction involves two steps :
- The first step is a Cα–H hydroxylation on the N-substituent to form a carbinolaniline complex .
- The second step is a decomposition of the carbinolaniline to yield cyclopropanone (or formaldehyde) and N-methylaniline (or N-cyclopropylaniline) .
Biochemical Pathways
The biochemical pathway affected by this compound is the cytochrome P450 metabolic pathway . The compound’s interaction with cytochrome P450 leads to changes in the metabolism of organic substances, impacting various downstream effects.
Result of Action
The result of the compound’s action is the formation of cyclopropanone (or formaldehyde) and N-methylaniline (or N-cyclopropylaniline) . These products are formed after the decomposition of the carbinolaniline complex .
Action Environment
The environment significantly influences the action of this compound. The environmental effect switches the regioselectivity of the reaction from a competition between N-decyclopropylation and N-demethylation to a clear preference for N-demethylation . This preference is consistent with former experimental studies .
Biochemical Analysis
Biochemical Properties
3-Cyclopropyl-N-methylaniline interacts with various enzymes and proteins in biochemical reactions . For instance, it has been found to be involved in reactions catalyzed by the enzyme cytochrome P450 . The nature of these interactions is complex and involves multiple steps, including a Cα–H hydroxylation on the N-substituent to form a carbinolaniline complex .
Cellular Effects
The effects of this compound on cells and cellular processes are diverse. It influences cell function by interacting with various cell signaling pathways, gene expression mechanisms, and cellular metabolism processes .
Molecular Mechanism
The molecular mechanism of action of this compound involves its binding interactions with biomolecules, enzyme inhibition or activation, and changes in gene expression . For example, it undergoes N-dealkylation catalyzed by cytochrome P450, a process that involves two steps: a Cα–H hydroxylation on the N-substituent to form a carbinolaniline complex, and a subsequent decomposition of the carbinolaniline .
Metabolic Pathways
This compound is involved in several metabolic pathways. It interacts with enzymes such as cytochrome P450 and undergoes processes like N-dealkylation
Preparation Methods
Synthetic Routes and Reaction Conditions
One common method for synthesizing 3-Cyclopropyl-N-methylaniline involves a multistep process starting with a Friedel-Crafts acylation, followed by a conversion from the acyl group to an alkane, and finally a nitration . The specific reaction conditions for each step may vary, but typically involve the use of catalysts and reagents such as aluminum chloride for the Friedel-Crafts acylation, and reducing agents for the conversion to an alkane.
Industrial Production Methods
Industrial production methods for this compound are not well-documented in the literature. large-scale synthesis would likely involve optimization of the laboratory-scale methods mentioned above, with a focus on maximizing yield and minimizing costs. This could include the use of continuous flow reactors and automated systems to streamline the process.
Chemical Reactions Analysis
Types of Reactions
3-Cyclopropyl-N-methylaniline undergoes various chemical reactions, including:
Oxidation: The compound can be oxidized to form corresponding N-oxides.
Reduction: Reduction reactions can convert it back to simpler amines.
Substitution: Electrophilic aromatic substitution reactions can introduce various substituents onto the benzene ring.
Common Reagents and Conditions
Oxidation: Common oxidizing agents include hydrogen peroxide and peracids.
Reduction: Reducing agents such as lithium aluminum hydride (LiAlH4) or sodium borohydride (NaBH4) are typically used.
Substitution: Reagents like halogens (e.g., chlorine, bromine) and nitrating agents (e.g., nitric acid) are used under acidic conditions.
Major Products Formed
Oxidation: N-oxides of this compound.
Reduction: Simpler amines such as N-methylaniline and cyclopropylamine.
Substitution: Various substituted derivatives depending on the reagents used.
Scientific Research Applications
3-Cyclopropyl-N-methylaniline has several applications in scientific research:
Chemistry: It is used as a building block in organic synthesis, particularly in the preparation of chiral cyclopropyl derivatives.
Biology: The compound is studied for its interactions with enzymes such as cytochrome P450, which catalyzes its N-dealkylation.
Industry: Used in the synthesis of advanced materials and specialty chemicals.
Comparison with Similar Compounds
Similar Compounds
N-Methylaniline: Similar structure but lacks the cyclopropyl group.
Cyclopropylamine: Contains the cyclopropyl group but lacks the aromatic ring.
Aniline: The parent compound without any substituents on the nitrogen.
Uniqueness
3-Cyclopropyl-N-methylaniline is unique due to the presence of both the cyclopropyl group and the methyl group on the nitrogen atom. This combination imparts distinct chemical properties and reactivity, making it valuable in various research applications.
Properties
IUPAC Name |
3-cyclopropyl-N-methylaniline |
Source
|
---|---|---|
Details | Computed by LexiChem 2.6.6 (PubChem release 2019.06.18) | |
Source | PubChem | |
URL | https://pubchem.ncbi.nlm.nih.gov | |
Description | Data deposited in or computed by PubChem | |
InChI |
InChI=1S/C10H13N/c1-11-10-4-2-3-9(7-10)8-5-6-8/h2-4,7-8,11H,5-6H2,1H3 |
Source
|
Details | Computed by InChI 1.0.5 (PubChem release 2019.06.18) | |
Source | PubChem | |
URL | https://pubchem.ncbi.nlm.nih.gov | |
Description | Data deposited in or computed by PubChem | |
InChI Key |
ZUPIODAOUBVVGZ-UHFFFAOYSA-N |
Source
|
Details | Computed by InChI 1.0.5 (PubChem release 2019.06.18) | |
Source | PubChem | |
URL | https://pubchem.ncbi.nlm.nih.gov | |
Description | Data deposited in or computed by PubChem | |
Canonical SMILES |
CNC1=CC=CC(=C1)C2CC2 |
Source
|
Details | Computed by OEChem 2.1.5 (PubChem release 2019.06.18) | |
Source | PubChem | |
URL | https://pubchem.ncbi.nlm.nih.gov | |
Description | Data deposited in or computed by PubChem | |
Molecular Formula |
C10H13N |
Source
|
Details | Computed by PubChem 2.1 (PubChem release 2019.06.18) | |
Source | PubChem | |
URL | https://pubchem.ncbi.nlm.nih.gov | |
Description | Data deposited in or computed by PubChem | |
Molecular Weight |
147.22 g/mol |
Source
|
Details | Computed by PubChem 2.1 (PubChem release 2021.05.07) | |
Source | PubChem | |
URL | https://pubchem.ncbi.nlm.nih.gov | |
Description | Data deposited in or computed by PubChem | |
Disclaimer and Information on In-Vitro Research Products
Please be aware that all articles and product information presented on BenchChem are intended solely for informational purposes. The products available for purchase on BenchChem are specifically designed for in-vitro studies, which are conducted outside of living organisms. In-vitro studies, derived from the Latin term "in glass," involve experiments performed in controlled laboratory settings using cells or tissues. It is important to note that these products are not categorized as medicines or drugs, and they have not received approval from the FDA for the prevention, treatment, or cure of any medical condition, ailment, or disease. We must emphasize that any form of bodily introduction of these products into humans or animals is strictly prohibited by law. It is essential to adhere to these guidelines to ensure compliance with legal and ethical standards in research and experimentation.