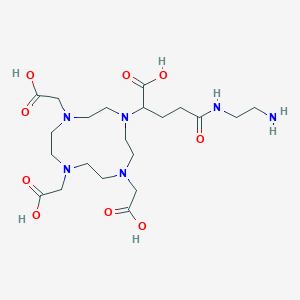
NH2-DOTA-GA
Overview
Description
Chemical Structure: NH2-DOTA-GA (C₂₁H₃₈N₆O₉, MW 518.56) is a bifunctional chelator composed of two key components:
- DOTA (1,4,7,10-tetraazacyclododecane-1,4,7,10-tetraacetic acid): A macrocyclic ligand known for forming stable complexes with metal ions (e.g., Gd³⁺, Zr⁴⁺, Lu³⁺) .
- Glycyrrhetinic Acid (GA): A triterpenoid derivative from licorice root, providing hydrophobicity and reactivity toward electrophilic reagents .
Preparation Methods
The synthesis of NH2-DOTA-GA involves a combination of solid-phase and solution-phase synthesis strategiesThe reaction conditions often involve the use of protective groups to ensure selective reactions at specific sites on the molecule .
For industrial production, the synthesis can be scaled up by optimizing the reaction conditions and using automated synthesis equipment. This ensures high yield and purity of the final product .
Chemical Reactions Analysis
NH2-DOTA-GA undergoes various chemical reactions, including complexation with metal ions, oxidation, and substitution reactions. The complexation with metal ions such as gallium-68 and lutetium-177 is particularly significant in its applications in nuclear medicine. The reaction conditions for complexation typically involve the use of buffers such as HEPES and ammonium acetate, with reaction temperatures around 95°C .
Scientific Research Applications
Chemical Properties and Mechanism of Action
Chemical Structure:
- Chemical Formula: C21H38N6O9
- Molecular Weight: 518.56 g/mol
- CAS Number: 1639843-65-4
NH2-DOTA-GA features an amino group that enhances its reactivity, allowing for effective conjugation with biomolecules such as peptides and antibodies. Its ability to chelate metal ions like Gadolinium (Gd) and Lutetium (Lu) is crucial for its applications in imaging and therapy .
Mechanism of Action:
- Chelation: this compound binds to metal ions, forming stable complexes that can be used for imaging or therapeutic purposes.
- Bioconjugation: The compound can be conjugated to various biomolecules, enabling targeted delivery to specific tissues or tumors.
Radiopharmaceuticals
This compound is extensively used in nuclear medicine for radiolabeling peptides and antibodies. This application is particularly relevant for imaging techniques such as:
- Positron Emission Tomography (PET)
- Single-Photon Emission Computed Tomography (SPECT)
The compound's ability to form stable complexes with radiometals enhances the efficacy of these imaging modalities, allowing for precise localization of tumors .
Targeted Drug Delivery
The compound's chelating properties facilitate the conjugation of drugs to this compound, which can then be complexed with a metal radioisotope. This approach allows for targeted therapy, minimizing damage to healthy tissues while maximizing therapeutic effects on cancer cells. For instance, conjugating this compound with Lutetium-177 enables targeted radiation therapy in cancer treatment .
Bioconjugation Techniques
This compound serves as a key tool in bioconjugation strategies, enhancing the specificity and stability of therapeutic agents. It has been successfully used to create conjugates for:
- Imaging agents targeting specific receptors (e.g., PSMA in prostate cancer)
- Therapeutic agents aimed at specific tumor types
Case Study 1: Prostate Cancer Imaging
In a study involving PSMA-targeted imaging agents, this compound was conjugated to a peptide ligand. The resulting complex demonstrated enhanced tumor accumulation and improved imaging contrast in preclinical models, highlighting its potential in prostate cancer diagnostics .
Case Study 2: Targeted Therapy with Lutetium-177
Research on this compound conjugated with Lutetium-177 showed promising results in delivering targeted radiation therapy to tumor cells while sparing surrounding healthy tissue. This study emphasized the compound's role in improving therapeutic outcomes in cancer treatment .
Mechanism of Action
The mechanism of action of NH2-DOTA-GA involves its ability to chelate metal ions, forming stable complexes that can be used for imaging and therapeutic purposes. The molecular targets and pathways involved depend on the specific radiolabeled compound. For example, when conjugated with prostate-specific membrane antigen (PSMA) ligands, the radiolabeled complex targets PSMA-expressing cells, enabling the imaging and treatment of prostate cancer .
Comparison with Similar Compounds
Physical Properties :
Table 1: Structural and Functional Comparison
Key Findings :
Chelation Efficiency :
- This compound and p-NH2-Bn-DOTA exhibit strong Gd³⁺ binding, but p-NH2-Bn-DOTA’s benzyl group improves tissue penetration .
- DOTAGA-Anhydride shows superior thermodynamic stability for Lu³⁺ in radiotherapy .
Biodistribution and Targeting :
- This compound’s GA moiety may enhance tumor targeting via glycyrrhetinic acid receptors .
- NH2-C4-DOTA’s aliphatic chain improves bone affinity due to hydrophobic interactions .
Synthetic Challenges :
- Cu⁺ retention in DOTA derivatives (e.g., p-NH2-Bn-DOTA) can reduce functionality and cause toxicity .
- This compound avoids transition-metal catalysts during synthesis, minimizing unwanted byproducts .
Relaxivity in MRI :
- This compound’s rigid linker and high molecular weight contribute to higher relaxivity compared to smaller chelators like DOTAGA-Anhydride .
Biological Activity
NH2-DOTA-GA, a bifunctional chelator, plays a significant role in radiolabeling and bioconjugation applications in nuclear medicine and molecular imaging. This article delves into its biological activity, mechanisms of action, pharmacokinetics, and recent research findings.
This compound is primarily used for labeling peptides and proteins, enabling targeted delivery of therapeutic agents or imaging agents. Its mechanism involves:
- Chelation : The compound forms stable complexes with metal ions (e.g., Gallium-68, Lutetium-177) through its DOTA moiety, facilitating radiolabeling for imaging and therapy.
- Targeting : When conjugated to specific biomolecules, this compound enhances the targeting of cancer cells, particularly in prostate cancer models.
2. Pharmacokinetics
The pharmacokinetic profile of this compound is characterized by:
- High Specific Activity : It achieves nearly quantitative radiochemical yields when labeled with Gallium-68 or Lutetium-177.
- Biodistribution : Studies indicate significant accumulation in tumor sites, particularly in LNCaP-tumor-bearing models, which underscores its potential for targeted cancer therapies.
This compound interacts with various biomolecules, impacting cellular processes:
- Cellular Effects : Research demonstrates that this compound can influence cell signaling pathways and gene expression in cancer cells.
- Stability : The compound exhibits high metabolic stability in biological systems, making it suitable for long-term applications in imaging and therapy.
4. Case Studies and Research Findings
Recent studies highlight the efficacy of this compound in clinical and preclinical settings:
4.1 Clinical Applications
- A phase I study involving 68Ga-NODAGA-LM3 and 68Ga-DOTA-LM3 showed promising results in patients with well-differentiated neuroendocrine tumors (NETs), indicating favorable biodistribution and dosimetry profiles .
4.2 Preclinical Studies
- In vitro and in vivo evaluations of this compound conjugates demonstrated enhanced targeting capabilities and therapeutic effects against various cancer types. For instance, conjugation to PSMA ligands has shown significant promise in prostate cancer imaging and therapy .
5. Data Tables
The following table summarizes key findings from recent studies on this compound:
6. Conclusion
This compound serves as a versatile tool in the fields of nuclear medicine and molecular imaging due to its effective chelation properties and ability to target specific biomolecules. Ongoing research continues to explore its applications in targeted therapies and diagnostic imaging, with promising results across various studies.
Q & A
Basic Research Questions
Q. What are the optimal synthesis protocols for NH2-DOTA-GA, and how can purity be validated?
this compound synthesis typically involves conjugating the DOTA chelator to glycyrrhetinic acid (GA) via amine-reactive crosslinkers. Solid-phase peptide synthesis (SPPS) is commonly used, with purification via reverse-phase HPLC and characterization using mass spectrometry (MS) and nuclear magnetic resonance (NMR) . Purity validation requires ≥95% chromatographic homogeneity, assessed using UV-Vis or fluorescence detectors. Researchers should document reaction yields, solvent systems, and purification thresholds to ensure reproducibility .
Q. How do researchers experimentally determine the chelation efficiency of this compound with radionuclides (e.g., ^89Zr, ^64Cu)?
Chelation efficiency is measured using inductively coupled plasma mass spectrometry (ICP-MS) or radio-TLC. For example, incubate this compound with ^89Zr-oxalate at varying pH (6.0–7.4) and temperatures (25–37°C). Centrifuge to separate unbound isotopes, and quantify bound radioactivity. Stability assays in serum (e.g., fetal bovine serum) over 48–72 hours assess in vivo applicability .
Advanced Research Questions
Q. How should preclinical studies using this compound-radiolabeled antibodies address discrepancies in biodistribution data across animal models?
Contradictions often arise from differences in tumor xenograft models (e.g., subcutaneous vs. orthotopic), radionuclide half-life, or imaging timepoints. Mitigate this by:
- Standardizing tumor inoculation protocols (cell count, growth monitoring).
- Using immuno-PET/MRI co-registration to validate targeting specificity.
- Applying kinetic modeling (e.g., compartmental analysis) to compare uptake rates . Document all variables in supplementary materials for cross-study validation .
Q. What methodological strategies improve the reproducibility of this compound-based radiopharmaceuticals in multi-modal imaging studies?
- Co-labeling validation: Use dual-isotope labeling (e.g., ^89Zr for PET and Gd³⁺ for MRI) to confirm targeting consistency.
- Data normalization: Correct for attenuation and scatter in PET using CT/MRI anatomical data.
- Batch testing: Compare chelation efficiency across synthesis batches using standardized QC protocols (e.g., EDTA challenge assays) . Reference NIH guidelines for preclinical imaging to ensure compliance .
Q. How can researchers resolve contradictions between in vitro stability assays and in vivo clearance rates of this compound complexes?
In vitro assays may overlook physiological factors (e.g., enzymatic degradation, protein binding). To address this:
- Perform serum stability tests using species-matched sera (e.g., human vs. murine).
- Correlate in vitro stability (half-life) with in vivo SPECT/CT biodistribution data at 24/48/72 hours.
- Use knockout animal models to isolate metabolic pathways affecting clearance .
Q. Methodological Best Practices
- Data documentation: Maintain raw spectral data (NMR, MS), radio-TLC images, and animal study logs in FAIR-compliant repositories .
- Conflict resolution: Apply Bland-Altman analysis to quantify inter-lab variability in chelation efficiency measurements .
- Ethical compliance: Annotate third-party data sources (e.g., public radiopharmaceutical databases) and disclose AI tools used for image analysis .
Properties
IUPAC Name |
5-(2-aminoethylamino)-5-oxo-2-[4,7,10-tris(carboxymethyl)-1,4,7,10-tetrazacyclododec-1-yl]pentanoic acid | |
---|---|---|
Details | Computed by LexiChem 2.6.6 (PubChem release 2019.06.18) | |
Source | PubChem | |
URL | https://pubchem.ncbi.nlm.nih.gov | |
Description | Data deposited in or computed by PubChem | |
InChI |
InChI=1S/C21H38N6O9/c22-3-4-23-17(28)2-1-16(21(35)36)27-11-9-25(14-19(31)32)7-5-24(13-18(29)30)6-8-26(10-12-27)15-20(33)34/h16H,1-15,22H2,(H,23,28)(H,29,30)(H,31,32)(H,33,34)(H,35,36) | |
Details | Computed by InChI 1.0.5 (PubChem release 2019.06.18) | |
Source | PubChem | |
URL | https://pubchem.ncbi.nlm.nih.gov | |
Description | Data deposited in or computed by PubChem | |
InChI Key |
IIKWGTMGEYTNCQ-UHFFFAOYSA-N | |
Details | Computed by InChI 1.0.5 (PubChem release 2019.06.18) | |
Source | PubChem | |
URL | https://pubchem.ncbi.nlm.nih.gov | |
Description | Data deposited in or computed by PubChem | |
Canonical SMILES |
C1CN(CCN(CCN(CCN1CC(=O)O)CC(=O)O)C(CCC(=O)NCCN)C(=O)O)CC(=O)O | |
Details | Computed by OEChem 2.1.5 (PubChem release 2019.06.18) | |
Source | PubChem | |
URL | https://pubchem.ncbi.nlm.nih.gov | |
Description | Data deposited in or computed by PubChem | |
Molecular Formula |
C21H38N6O9 | |
Details | Computed by PubChem 2.1 (PubChem release 2019.06.18) | |
Source | PubChem | |
URL | https://pubchem.ncbi.nlm.nih.gov | |
Description | Data deposited in or computed by PubChem | |
Molecular Weight |
518.6 g/mol | |
Details | Computed by PubChem 2.1 (PubChem release 2021.05.07) | |
Source | PubChem | |
URL | https://pubchem.ncbi.nlm.nih.gov | |
Description | Data deposited in or computed by PubChem | |
Retrosynthesis Analysis
AI-Powered Synthesis Planning: Our tool employs the Template_relevance Pistachio, Template_relevance Bkms_metabolic, Template_relevance Pistachio_ringbreaker, Template_relevance Reaxys, Template_relevance Reaxys_biocatalysis model, leveraging a vast database of chemical reactions to predict feasible synthetic routes.
One-Step Synthesis Focus: Specifically designed for one-step synthesis, it provides concise and direct routes for your target compounds, streamlining the synthesis process.
Accurate Predictions: Utilizing the extensive PISTACHIO, BKMS_METABOLIC, PISTACHIO_RINGBREAKER, REAXYS, REAXYS_BIOCATALYSIS database, our tool offers high-accuracy predictions, reflecting the latest in chemical research and data.
Strategy Settings
Precursor scoring | Relevance Heuristic |
---|---|
Min. plausibility | 0.01 |
Model | Template_relevance |
Template Set | Pistachio/Bkms_metabolic/Pistachio_ringbreaker/Reaxys/Reaxys_biocatalysis |
Top-N result to add to graph | 6 |
Feasible Synthetic Routes
Disclaimer and Information on In-Vitro Research Products
Please be aware that all articles and product information presented on BenchChem are intended solely for informational purposes. The products available for purchase on BenchChem are specifically designed for in-vitro studies, which are conducted outside of living organisms. In-vitro studies, derived from the Latin term "in glass," involve experiments performed in controlled laboratory settings using cells or tissues. It is important to note that these products are not categorized as medicines or drugs, and they have not received approval from the FDA for the prevention, treatment, or cure of any medical condition, ailment, or disease. We must emphasize that any form of bodily introduction of these products into humans or animals is strictly prohibited by law. It is essential to adhere to these guidelines to ensure compliance with legal and ethical standards in research and experimentation.