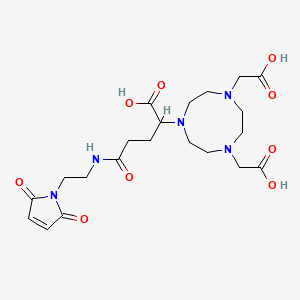
Maleimide-NODA-GA
Overview
Description
Maleimide-NODA-GA is a bifunctional chelator used in radiopharmaceuticals, particularly for positron emission tomography (PET) imaging. It is designed to bind with gallium-68, a radionuclide, to form stable complexes that can be used for imaging purposes. The compound is known for its high stability and specificity in targeting certain proteins or receptors in biological systems .
Mechanism of Action
Target of Action
Maleimide-NODA-GA is primarily used for labeling peptides and antibodies . It specifically reacts with the sulfhydryl group of cysteine residues . This allows the compound to form stable bioconjugates while preserving the functionality and specificity of the biomolecules .
Mode of Action
The mode of action of this compound involves the formation of a covalent bond with the sulfhydryl group of cysteine residues . This reaction results in the labeling of peptides and antibodies, which can be used for various research and diagnostic applications .
Pharmacokinetics
The pharmacokinetics of this compound, such as its absorption, distribution, metabolism, and excretion (ADME) properties, can be tailored by optimizing the size, charge, hydrophobicity, or targeting ligand of the bioconjugates . For instance, a study on a variant of a 68Ga-labeled probe showed rapid clearance of the probe via the renal route, which corresponded to low background signals in PET images .
Result of Action
The result of this compound’s action is the successful labeling of peptides and antibodies. This enables the visualization and tracking of these biomolecules in biological systems, which can be crucial in research and diagnostic applications. For example, a study demonstrated efficient delineation of a tumor using a 68Ga-labeled probe .
Action Environment
The action, efficacy, and stability of this compound can be influenced by various environmental factors. These include the pH and temperature of the reaction environment, as well as the presence of other reactive groups. It is recommended to handle the compound under dry conditions and avoid frequent dissolution and freeze-drying . The compound should be brought to room temperature slowly from the refrigerator before opening the container .
Biochemical Analysis
Biochemical Properties
Maleimide-NODA-GA has a specific reactivity towards the SH group such as cysteine . This allows it to interact with proteins and peptides that contain this group. The interaction occurs through a Michael addition reaction, where the maleimide acts as the Michael acceptor and the thiolate, present in the cysteine, acts as the Michael donor .
Cellular Effects
The cellular effects of this compound are primarily related to its ability to label peptides and antibodies This labeling can influence various cellular processes, including cell signaling pathways and gene expression
Molecular Mechanism
The molecular mechanism of action of this compound involves its reaction with cysteine groups on proteins. This reaction is a type of Michael addition, where the maleimide acts as the Michael acceptor and the thiolate (from the cysteine) is the Michael donor . This reaction leads to the formation of a thiosuccinimide complex, which can undergo hydrolysis to form a stable succinamic acid thioether .
Temporal Effects in Laboratory Settings
It is known that the maleimide-thiol reaction is slowly reversible under certain conditions . Despite this, the maleimide moiety itself is relatively stable to degradation .
Dosage Effects in Animal Models
A study using a variant of a 68Ga-labeled probe developed with this compound for PET imaging demonstrated its safety and feasibility in immunocompetent mice and non-human primates .
Metabolic Pathways
Given its reactivity with cysteine, it may interact with metabolic pathways involving proteins and peptides that contain this amino acid .
Preparation Methods
Synthetic Routes and Reaction Conditions
The synthesis of Maleimide-NODA-GA involves the reaction of maleimide with NODA-GA (1,4,7-triazacyclononane,1-glutaric acid-4,7-acetic acid). The process typically includes the following steps:
Preparation of NODA-GA: NODA-GA is synthesized by reacting 1,4,7-triazacyclononane with glutaric acid and acetic acid.
Conjugation with Maleimide: The maleimide group is introduced by reacting NODA-GA with maleimide under mild conditions, usually at room temperature and in the presence of a catalyst.
Industrial Production Methods
Industrial production of this compound follows similar synthetic routes but on a larger scale. The process is optimized for high yield and purity, often involving automated synthesis and purification techniques to ensure consistency and quality .
Chemical Reactions Analysis
Types of Reactions
Maleimide-NODA-GA primarily undergoes addition reactions, particularly the thiol-maleimide reaction, which is a type of click chemistry. This reaction involves the addition of a thiol group to the maleimide moiety, forming a stable thiosuccinimide product .
Common Reagents and Conditions
Thiol Compounds: Common thiol reagents include cysteine and glutathione.
Reaction Conditions: The reaction typically occurs at a pH range of 6.5 to 7.5, with optimal conditions around pH 7.0.
Major Products
The major product of the thiol-maleimide reaction is a thiosuccinimide derivative, which is highly stable and suitable for further applications in bioconjugation and imaging .
Scientific Research Applications
Maleimide-NODA-GA has a wide range of applications in scientific research, particularly in the fields of chemistry, biology, medicine, and industry.
Chemistry: Used as a chelating agent for radiolabeling proteins and peptides with gallium-68 for PET imaging.
Biology: Employed in the study of protein-protein interactions and the mapping of biological pathways.
Medicine: Utilized in the development of diagnostic imaging agents for cancer and other diseases.
Comparison with Similar Compounds
Maleimide-NODA-GA is compared with other chelating agents such as DOTA (1,4,7,10-tetraazacyclododecane-1,4,7,10-tetraacetic acid) and NOTA (1,4,7-triazacyclononane-1,4,7-triacetic acid).
Similar Compounds
- DOTA
- NOTA
- TRAP (triazacyclononane-phosphinate)
This compound stands out due to its high stability, specificity, and efficiency in forming complexes with gallium-68, making it a valuable tool in the field of radiopharmaceuticals and diagnostic imaging.
Properties
IUPAC Name |
2-[4,7-bis(carboxymethyl)-1,4,7-triazonan-1-yl]-5-[2-(2,5-dioxopyrrol-1-yl)ethylamino]-5-oxopentanoic acid | |
---|---|---|
Details | Computed by Lexichem TK 2.7.0 (PubChem release 2021.05.07) | |
Source | PubChem | |
URL | https://pubchem.ncbi.nlm.nih.gov | |
Description | Data deposited in or computed by PubChem | |
InChI |
InChI=1S/C21H31N5O9/c27-16(22-5-6-26-17(28)3-4-18(26)29)2-1-15(21(34)35)25-11-9-23(13-19(30)31)7-8-24(10-12-25)14-20(32)33/h3-4,15H,1-2,5-14H2,(H,22,27)(H,30,31)(H,32,33)(H,34,35) | |
Details | Computed by InChI 1.0.6 (PubChem release 2021.05.07) | |
Source | PubChem | |
URL | https://pubchem.ncbi.nlm.nih.gov | |
Description | Data deposited in or computed by PubChem | |
InChI Key |
SJUXYJQNMNJVFX-UHFFFAOYSA-N | |
Details | Computed by InChI 1.0.6 (PubChem release 2021.05.07) | |
Source | PubChem | |
URL | https://pubchem.ncbi.nlm.nih.gov | |
Description | Data deposited in or computed by PubChem | |
Canonical SMILES |
C1CN(CCN(CCN1CC(=O)O)C(CCC(=O)NCCN2C(=O)C=CC2=O)C(=O)O)CC(=O)O | |
Details | Computed by OEChem 2.3.0 (PubChem release 2021.05.07) | |
Source | PubChem | |
URL | https://pubchem.ncbi.nlm.nih.gov | |
Description | Data deposited in or computed by PubChem | |
Molecular Formula |
C21H31N5O9 | |
Details | Computed by PubChem 2.1 (PubChem release 2021.05.07) | |
Source | PubChem | |
URL | https://pubchem.ncbi.nlm.nih.gov | |
Description | Data deposited in or computed by PubChem | |
Molecular Weight |
497.5 g/mol | |
Details | Computed by PubChem 2.1 (PubChem release 2021.05.07) | |
Source | PubChem | |
URL | https://pubchem.ncbi.nlm.nih.gov | |
Description | Data deposited in or computed by PubChem | |
Retrosynthesis Analysis
AI-Powered Synthesis Planning: Our tool employs the Template_relevance Pistachio, Template_relevance Bkms_metabolic, Template_relevance Pistachio_ringbreaker, Template_relevance Reaxys, Template_relevance Reaxys_biocatalysis model, leveraging a vast database of chemical reactions to predict feasible synthetic routes.
One-Step Synthesis Focus: Specifically designed for one-step synthesis, it provides concise and direct routes for your target compounds, streamlining the synthesis process.
Accurate Predictions: Utilizing the extensive PISTACHIO, BKMS_METABOLIC, PISTACHIO_RINGBREAKER, REAXYS, REAXYS_BIOCATALYSIS database, our tool offers high-accuracy predictions, reflecting the latest in chemical research and data.
Strategy Settings
Precursor scoring | Relevance Heuristic |
---|---|
Min. plausibility | 0.01 |
Model | Template_relevance |
Template Set | Pistachio/Bkms_metabolic/Pistachio_ringbreaker/Reaxys/Reaxys_biocatalysis |
Top-N result to add to graph | 6 |
Feasible Synthetic Routes
Disclaimer and Information on In-Vitro Research Products
Please be aware that all articles and product information presented on BenchChem are intended solely for informational purposes. The products available for purchase on BenchChem are specifically designed for in-vitro studies, which are conducted outside of living organisms. In-vitro studies, derived from the Latin term "in glass," involve experiments performed in controlled laboratory settings using cells or tissues. It is important to note that these products are not categorized as medicines or drugs, and they have not received approval from the FDA for the prevention, treatment, or cure of any medical condition, ailment, or disease. We must emphasize that any form of bodily introduction of these products into humans or animals is strictly prohibited by law. It is essential to adhere to these guidelines to ensure compliance with legal and ethical standards in research and experimentation.