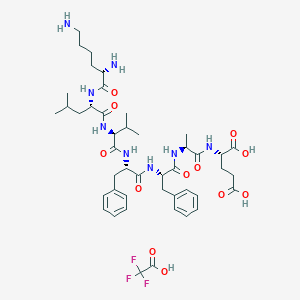
Amyloid b-Protein (16-22) Trifluoroacetate
Overview
Description
Amyloid b-Protein (16-22) Trifluoroacetate is a peptide fragment derived from the amyloid b-protein precursor. This compound has garnered significant attention due to its involvement in the pathogenesis of Alzheimer’s disease. The peptide sequence consists of seven amino acids and is known for its ability to form amyloid fibrils, which are associated with neurodegenerative conditions.
Mechanism of Action
Target of Action
Amyloid β-Protein (16-22) Trifluoroacetate is a fragment of the larger Amyloid β-Protein . The primary targets of this compound are amyloid plaques found in the brain, which are a hallmark of neurodegenerative diseases .
Mode of Action
The compound interacts with its targets by binding to the amyloid plaques. This interaction can lead to changes in the structure and function of the plaques . The process can be accelerated by hydrophobic interfaces such as the cell membrane interface and the air−water interface .
Biochemical Pathways
The compound affects the assembly and fibrillation of Aβ peptides, which are vital for various neurodegenerative diseases . The fibrilization process can be promoted by increasing hydrophobic contacts .
Result of Action
The molecular and cellular effects of the compound’s action are complex and depend on a variety of factors. It is known that the compound can influence the structure and function of amyloid plaques, potentially affecting the progression of neurodegenerative diseases .
Action Environment
Environmental factors can influence the compound’s action, efficacy, and stability. For example, the presence of hydrophobic interfaces can accelerate the compound’s interaction with its targets . Additionally, the pH of the environment can affect the structure of the compound and its ability to interact with its targets .
Biochemical Analysis
Biochemical Properties
Amyloid b-Protein (16-22) Trifluoroacetate plays a crucial role in biochemical reactions, particularly in the formation of amyloid fibrils. This peptide interacts with various enzymes, proteins, and other biomolecules. One of the key interactions is with hydrophobic macromolecular crowders, which can influence the aggregation process of the peptide. The presence of hydrophobic crowders can increase the fibrillation lag time or even prevent the formation of fibrils altogether . Additionally, this compound can interact with other amyloid peptides, promoting the formation of larger oligomers, fibrils, and plaques .
Cellular Effects
This compound has significant effects on various types of cells and cellular processes. In the context of Alzheimer’s disease, this peptide aggregates in the brain, forming structures that cause cognitive impairment and memory loss . The aggregation process is influenced by the presence of other macromolecular species, which can exert forces on the proteins. These interactions can alter cell signaling pathways, gene expression, and cellular metabolism. For example, the presence of hydrophobic crowders can reduce the antiparallel b-sheet content of fibrils, affecting the overall structure and function of the aggregates .
Molecular Mechanism
The mechanism of action of this compound involves its ability to form amyloid fibrils through a process of nucleation and aggregation. At the molecular level, this peptide can undergo conformational changes, influenced by factors such as pH and the presence of hydrophobic interfaces . At neutral pH, the peptides adopt a less folded, bending motif by forming intra-hydrogen bonds. At acidic pH, the peptides refold into extended b-strand fibril conformation, promoting their macroscopic assembly . The protonation of acidic glutamic acid side chains reduces hydrogen bonds, driving the conformational transition.
Temporal Effects in Laboratory Settings
In laboratory settings, the effects of this compound can change over time. The stability and degradation of this peptide are influenced by various factors, including pH and the presence of other macromolecular species. Over time, the peptide can form larger aggregates, such as oligomers and fibrils, which can have long-term effects on cellular function. The stability of the peptide is also affected by its interactions with hydrophobic interfaces, which can accelerate the aggregation process .
Dosage Effects in Animal Models
The effects of this compound vary with different dosages in animal models. At lower dosages, the peptide may not form significant aggregates, while at higher dosages, it can lead to the formation of amyloid fibrils and plaques. These aggregates can cause neuroinflammation and other adverse effects in animal models . The threshold effects observed in these studies indicate that there is a critical concentration of the peptide required for fibril formation.
Metabolic Pathways
This compound is involved in various metabolic pathways, particularly those related to protein aggregation and amyloid formation. The peptide can interact with enzymes and cofactors that influence its aggregation process. For example, the presence of hydrophobic crowders can alter the secondary structure of the peptide, preventing the formation of ordered b-sheets or fibrils . Additionally, the peptide can undergo conformational changes that affect its metabolic flux and metabolite levels .
Transport and Distribution
Within cells and tissues, this compound is transported and distributed through interactions with transporters and binding proteins. These interactions can influence the localization and accumulation of the peptide. For example, the peptide can interact with ionic liquids, which can affect its conformational dynamics and stability . The transport and distribution of the peptide are also influenced by its interactions with hydrophobic interfaces, which can promote its aggregation and localization in specific cellular compartments .
Subcellular Localization
The subcellular localization of this compound is influenced by various factors, including targeting signals and post-translational modifications. The peptide can be directed to specific compartments or organelles, where it can exert its effects on cellular function. For example, the peptide can localize to the cell membrane or the air-water interface, where it can form amyloid fibrils . The localization of the peptide can also be influenced by its interactions with other biomolecules, which can affect its activity and function .
Preparation Methods
Synthetic Routes and Reaction Conditions
The synthesis of Amyloid b-Protein (16-22) Trifluoroacetate typically involves solid-phase peptide synthesis (SPPS). This method allows for the sequential addition of amino acids to a growing peptide chain anchored to a solid resin. The process includes the following steps:
Coupling: The first amino acid is attached to the resin.
Deprotection: The protecting group on the amino acid is removed.
Elongation: Subsequent amino acids are added one by one through coupling reactions.
Cleavage: The completed peptide is cleaved from the resin and purified.
Industrial Production Methods
Industrial production of this compound follows similar principles as laboratory synthesis but on a larger scale. Automated peptide synthesizers are often used to increase efficiency and yield. The process involves rigorous quality control measures to ensure the purity and consistency of the final product .
Chemical Reactions Analysis
Types of Reactions
Amyloid b-Protein (16-22) Trifluoroacetate can undergo various chemical reactions, including:
Oxidation: This reaction can lead to the formation of disulfide bonds between cysteine residues.
Reduction: Reduction reactions can break disulfide bonds, leading to changes in the peptide’s conformation.
Substitution: Amino acid residues can be substituted to study the effects on peptide aggregation and fibril formation.
Common Reagents and Conditions
Common reagents used in these reactions include:
Oxidizing agents: Hydrogen peroxide, iodine.
Reducing agents: Dithiothreitol (DTT), beta-mercaptoethanol.
Substitution reagents: Amino acid derivatives, coupling agents like HBTU (O-Benzotriazole-N,N,N’,N’-tetramethyl-uronium-hexafluoro-phosphate).
Major Products Formed
The major products formed from these reactions include modified peptides with altered structural and functional properties. These modifications can affect the peptide’s ability to form amyloid fibrils and its interaction with other molecules .
Scientific Research Applications
Amyloid b-Protein (16-22) Trifluoroacetate has a wide range of scientific research applications:
Chemistry: Used to study peptide synthesis, aggregation, and fibril formation.
Biology: Investigated for its role in protein misfolding and aggregation in neurodegenerative diseases.
Medicine: Explored as a potential target for therapeutic interventions in Alzheimer’s disease.
Industry: Utilized in the development of diagnostic tools and assays for detecting amyloid fibrils.
Comparison with Similar Compounds
Similar Compounds
Amyloid b-Protein (1-42) Trifluoroacetate: A longer peptide fragment with similar amyloidogenic properties.
Amyloid b-Protein (25-35) Trifluoroacetate: Another peptide fragment known for its role in amyloid fibril formation.
Uniqueness
Amyloid b-Protein (16-22) Trifluoroacetate is unique due to its specific sequence and its ability to form amyloid fibrils rapidly. This makes it a valuable model for studying the mechanisms of amyloid formation and for developing therapeutic strategies to combat amyloid-related diseases.
Properties
IUPAC Name |
(2S)-2-[[(2S)-2-[[(2S)-2-[[(2S)-2-[[(2S)-2-[[(2S)-2-[[(2S)-2,6-diaminohexanoyl]amino]-4-methylpentanoyl]amino]-3-methylbutanoyl]amino]-3-phenylpropanoyl]amino]-3-phenylpropanoyl]amino]propanoyl]amino]pentanedioic acid;2,2,2-trifluoroacetic acid | |
---|---|---|
Details | Computed by LexiChem 2.6.6 (PubChem release 2019.06.18) | |
Source | PubChem | |
URL | https://pubchem.ncbi.nlm.nih.gov | |
Description | Data deposited in or computed by PubChem | |
InChI |
InChI=1S/C43H64N8O10.C2HF3O2/c1-25(2)22-32(48-38(55)30(45)18-12-13-21-44)41(58)51-36(26(3)4)42(59)50-34(24-29-16-10-7-11-17-29)40(57)49-33(23-28-14-8-6-9-15-28)39(56)46-27(5)37(54)47-31(43(60)61)19-20-35(52)53;3-2(4,5)1(6)7/h6-11,14-17,25-27,30-34,36H,12-13,18-24,44-45H2,1-5H3,(H,46,56)(H,47,54)(H,48,55)(H,49,57)(H,50,59)(H,51,58)(H,52,53)(H,60,61);(H,6,7)/t27-,30-,31-,32-,33-,34-,36-;/m0./s1 | |
Details | Computed by InChI 1.0.5 (PubChem release 2019.06.18) | |
Source | PubChem | |
URL | https://pubchem.ncbi.nlm.nih.gov | |
Description | Data deposited in or computed by PubChem | |
InChI Key |
WMQMXRMQUXWHCC-YJVMEOIXSA-N | |
Details | Computed by InChI 1.0.5 (PubChem release 2019.06.18) | |
Source | PubChem | |
URL | https://pubchem.ncbi.nlm.nih.gov | |
Description | Data deposited in or computed by PubChem | |
Canonical SMILES |
CC(C)CC(C(=O)NC(C(C)C)C(=O)NC(CC1=CC=CC=C1)C(=O)NC(CC2=CC=CC=C2)C(=O)NC(C)C(=O)NC(CCC(=O)O)C(=O)O)NC(=O)C(CCCCN)N.C(=O)(C(F)(F)F)O | |
Details | Computed by OEChem 2.1.5 (PubChem release 2019.06.18) | |
Source | PubChem | |
URL | https://pubchem.ncbi.nlm.nih.gov | |
Description | Data deposited in or computed by PubChem | |
Isomeric SMILES |
C[C@@H](C(=O)N[C@@H](CCC(=O)O)C(=O)O)NC(=O)[C@H](CC1=CC=CC=C1)NC(=O)[C@H](CC2=CC=CC=C2)NC(=O)[C@H](C(C)C)NC(=O)[C@H](CC(C)C)NC(=O)[C@H](CCCCN)N.C(=O)(C(F)(F)F)O | |
Details | Computed by OEChem 2.1.5 (PubChem release 2019.06.18) | |
Source | PubChem | |
URL | https://pubchem.ncbi.nlm.nih.gov | |
Description | Data deposited in or computed by PubChem | |
Molecular Formula |
C45H65F3N8O12 | |
Details | Computed by PubChem 2.1 (PubChem release 2019.06.18) | |
Source | PubChem | |
URL | https://pubchem.ncbi.nlm.nih.gov | |
Description | Data deposited in or computed by PubChem | |
Molecular Weight |
967.0 g/mol | |
Details | Computed by PubChem 2.1 (PubChem release 2021.05.07) | |
Source | PubChem | |
URL | https://pubchem.ncbi.nlm.nih.gov | |
Description | Data deposited in or computed by PubChem | |
Disclaimer and Information on In-Vitro Research Products
Please be aware that all articles and product information presented on BenchChem are intended solely for informational purposes. The products available for purchase on BenchChem are specifically designed for in-vitro studies, which are conducted outside of living organisms. In-vitro studies, derived from the Latin term "in glass," involve experiments performed in controlled laboratory settings using cells or tissues. It is important to note that these products are not categorized as medicines or drugs, and they have not received approval from the FDA for the prevention, treatment, or cure of any medical condition, ailment, or disease. We must emphasize that any form of bodily introduction of these products into humans or animals is strictly prohibited by law. It is essential to adhere to these guidelines to ensure compliance with legal and ethical standards in research and experimentation.