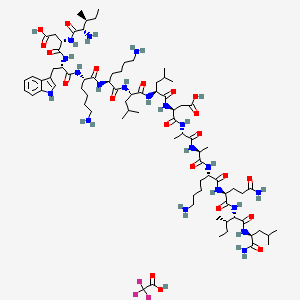
Polybia-MP1 Trifluoroacetate
- Click on QUICK INQUIRY to receive a quote from our team of experts.
- With the quality product at a COMPETITIVE price, you can focus more on your research.
Overview
Description
Polybia-MP1 is an antimicrobial peptide that has been isolated from the venom of the vespid wasp Polybia paulista . It is an amphipathic tetradecapeptide with potent antimicrobial activity and high selectivity for bacterial membranes . It has been shown to inhibit the growth of antibiotic-resistant Pseudomonas aeruginosa .
Synthesis Analysis
The synthesis of Polybia-MP1 involves obtaining it with a free N terminal and a C terminal amide group after cleavage from Rink amide MBHA resin . After HPLC purification, the peptide has been reported to have > 90% purity .Molecular Structure Analysis
Polybia-MP1 is characterized by its unique properties such as low net positive charge, high helical content, and a high proportion of anionic residues compared to other peptides in the Mastoparan family .Physical And Chemical Properties Analysis
Polybia-MP1 is a 14 amino acid long peptide . It has a molecular weight of 1654.03 and its chemical formula is C₇₈H₁₃₂N₂₀O₁₉ .Scientific Research Applications
Cancer Research Applications
Selective Toxicity to Cancer Cells: Polybia-MP1 has been shown to selectively kill cancer cells without harming normal cells. This is achieved by the peptide’s interaction with lipids that are abnormally distributed on the surface of cancer cells, causing the cells to open up and die .
Mechanism of Action Characterization: Detailed mechanistic characterization of MP1 has been conducted using biophysical and biological approaches, providing insights into the molecular basis underlying cell sensitivity to MP1 .
Antibacterial Research Applications
Broad Antibacterial Spectrum: Polybia-MP1 displays a broad antibacterial spectrum, making it a potential candidate for treating various bacterial infections .
Low Toxicity to Human Cells: The peptide has low toxicity to human red blood cells and normal fibroblasts, which is crucial for its potential therapeutic use .
Effectiveness Against Antibiotic-Resistant Bacteria: Polybia-MP1 has shown effective antimicrobial activity against antibiotic-resistant Pseudomonas aeruginosa isolates obtained from mastitic cow milk samples, demonstrating its potential in combating antibiotic resistance .
Potential in Veterinary Medicine: The effectiveness of Polybia-MP1 against bacteria isolated from mastitic cow milk samples suggests its potential application in veterinary medicine, particularly in treating mastitis in cows .
Antifungal Research Applications
- Antifungal Properties In addition to its antibacterial activity, Polybia-MP1 also exhibits antifungal properties, expanding its potential therapeutic applications .
Biophysical Research Applications
Mechanism of Action
Target of Action
Polybia-MP1 Trifluoroacetate, a natural antimicrobial peptide, has been intensively studied due to its therapeutic potential . It exhibits potent antibacterial, antifungal, and anticancer properties . The primary targets of Polybia-MP1 are bacterial and cancer cells .
Mode of Action
Polybia-MP1 interacts with its targets primarily through membrane perturbation . It is an amphipathic tetradecapeptide, which means it has both hydrophilic and hydrophobic regions. This allows it to interact with the lipid bilayer of cell membranes, leading to membrane disruption .
Biochemical Pathways
This disruption could include interference with nutrient uptake, protein synthesis, or DNA replication, leading to cell death .
Pharmacokinetics
Its potent antimicrobial activity against antibiotic-resistant pseudomonas aeruginosa suggests that it has good bioavailability .
Result of Action
Polybia-MP1 has been shown to effectively inhibit the growth of antibiotic-resistant Pseudomonas aeruginosa . . This makes it a promising candidate for therapeutic applications.
Safety and Hazards
Future Directions
Given its potent antimicrobial activity and relatively low toxicity, Polybia-MP1 is being explored as a potential alternative to antibiotics for the treatment of diseases like mastitis in cattle . There is also ongoing research to develop this peptide to become more “druggable” by creating modified analogs with better pharmacological properties .
properties
IUPAC Name |
(3S)-4-[[(2S)-1-[[(2S)-1-[[(2S)-6-amino-1-[[(2S)-5-amino-1-[[(2S,3S)-1-[[(2S)-1-amino-4-methyl-1-oxopentan-2-yl]amino]-3-methyl-1-oxopentan-2-yl]amino]-1,5-dioxopentan-2-yl]amino]-1-oxohexan-2-yl]amino]-1-oxopropan-2-yl]amino]-1-oxopropan-2-yl]amino]-3-[[(2S)-2-[[(2S)-2-[[(2S)-6-amino-2-[[(2S)-6-amino-2-[[(2S)-2-[[(2S)-2-[[(2S,3S)-2-amino-3-methylpentanoyl]amino]-3-carboxypropanoyl]amino]-3-(1H-indol-3-yl)propanoyl]amino]hexanoyl]amino]hexanoyl]amino]-4-methylpentanoyl]amino]-4-methylpentanoyl]amino]-4-oxobutanoic acid;2,2,2-trifluoroacetic acid |
Source
|
---|---|---|
Details | Computed by Lexichem TK 2.7.0 (PubChem release 2021.05.07) | |
Source | PubChem | |
URL | https://pubchem.ncbi.nlm.nih.gov | |
Description | Data deposited in or computed by PubChem | |
InChI |
InChI=1S/C78H132N20O19.C2HF3O2/c1-13-43(9)63(83)77(116)97-59(38-62(102)103)76(115)95-57(36-47-39-85-49-24-16-15-23-48(47)49)75(114)90-51(26-18-21-31-80)69(108)89-52(27-19-22-32-81)70(109)93-55(34-41(5)6)73(112)94-56(35-42(7)8)74(113)96-58(37-61(100)101)72(111)87-45(11)66(105)86-46(12)67(106)88-50(25-17-20-30-79)68(107)91-53(28-29-60(82)99)71(110)98-64(44(10)14-2)78(117)92-54(65(84)104)33-40(3)4;3-2(4,5)1(6)7/h15-16,23-24,39-46,50-59,63-64,85H,13-14,17-22,25-38,79-81,83H2,1-12H3,(H2,82,99)(H2,84,104)(H,86,105)(H,87,111)(H,88,106)(H,89,108)(H,90,114)(H,91,107)(H,92,117)(H,93,109)(H,94,112)(H,95,115)(H,96,113)(H,97,116)(H,98,110)(H,100,101)(H,102,103);(H,6,7)/t43-,44-,45-,46-,50-,51-,52-,53-,54-,55-,56-,57-,58-,59-,63-,64-;/m0./s1 |
Source
|
Details | Computed by InChI 1.0.6 (PubChem release 2021.05.07) | |
Source | PubChem | |
URL | https://pubchem.ncbi.nlm.nih.gov | |
Description | Data deposited in or computed by PubChem | |
InChI Key |
LVLZKCHCKFKPQT-FLKJMJLMSA-N |
Source
|
Details | Computed by InChI 1.0.6 (PubChem release 2021.05.07) | |
Source | PubChem | |
URL | https://pubchem.ncbi.nlm.nih.gov | |
Description | Data deposited in or computed by PubChem | |
Canonical SMILES |
CCC(C)C(C(=O)NC(CC(=O)O)C(=O)NC(CC1=CNC2=CC=CC=C21)C(=O)NC(CCCCN)C(=O)NC(CCCCN)C(=O)NC(CC(C)C)C(=O)NC(CC(C)C)C(=O)NC(CC(=O)O)C(=O)NC(C)C(=O)NC(C)C(=O)NC(CCCCN)C(=O)NC(CCC(=O)N)C(=O)NC(C(C)CC)C(=O)NC(CC(C)C)C(=O)N)N.C(=O)(C(F)(F)F)O |
Source
|
Details | Computed by OEChem 2.3.0 (PubChem release 2021.05.07) | |
Source | PubChem | |
URL | https://pubchem.ncbi.nlm.nih.gov | |
Description | Data deposited in or computed by PubChem | |
Isomeric SMILES |
CC[C@H](C)[C@@H](C(=O)N[C@@H](CC(=O)O)C(=O)N[C@@H](CC1=CNC2=CC=CC=C21)C(=O)N[C@@H](CCCCN)C(=O)N[C@@H](CCCCN)C(=O)N[C@@H](CC(C)C)C(=O)N[C@@H](CC(C)C)C(=O)N[C@@H](CC(=O)O)C(=O)N[C@@H](C)C(=O)N[C@@H](C)C(=O)N[C@@H](CCCCN)C(=O)N[C@@H](CCC(=O)N)C(=O)N[C@@H]([C@@H](C)CC)C(=O)N[C@@H](CC(C)C)C(=O)N)N.C(=O)(C(F)(F)F)O |
Source
|
Details | Computed by OEChem 2.3.0 (PubChem release 2021.05.07) | |
Source | PubChem | |
URL | https://pubchem.ncbi.nlm.nih.gov | |
Description | Data deposited in or computed by PubChem | |
Molecular Formula |
C80H133F3N20O21 |
Source
|
Details | Computed by PubChem 2.1 (PubChem release 2021.05.07) | |
Source | PubChem | |
URL | https://pubchem.ncbi.nlm.nih.gov | |
Description | Data deposited in or computed by PubChem | |
Molecular Weight |
1768.0 g/mol |
Source
|
Details | Computed by PubChem 2.1 (PubChem release 2021.05.07) | |
Source | PubChem | |
URL | https://pubchem.ncbi.nlm.nih.gov | |
Description | Data deposited in or computed by PubChem | |
Product Name |
H-Ile-Asp-Trp-Lys-Lys-Leu-Leu-Asp-Ala-Ala-Lys-Gln-Ile-Leu-NH2.TFA |
Disclaimer and Information on In-Vitro Research Products
Please be aware that all articles and product information presented on BenchChem are intended solely for informational purposes. The products available for purchase on BenchChem are specifically designed for in-vitro studies, which are conducted outside of living organisms. In-vitro studies, derived from the Latin term "in glass," involve experiments performed in controlled laboratory settings using cells or tissues. It is important to note that these products are not categorized as medicines or drugs, and they have not received approval from the FDA for the prevention, treatment, or cure of any medical condition, ailment, or disease. We must emphasize that any form of bodily introduction of these products into humans or animals is strictly prohibited by law. It is essential to adhere to these guidelines to ensure compliance with legal and ethical standards in research and experimentation.