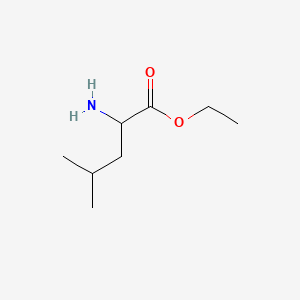
Ethyl 2-amino-4-methylpentanoate
- Click on QUICK INQUIRY to receive a quote from our team of experts.
- With the quality product at a COMPETITIVE price, you can focus more on your research.
Overview
Description
Preparation Methods
Synthetic Routes and Reaction Conditions: Ethyl 2-amino-4-methylpentanoate can be synthesized through the esterification of 2-amino-4-methylpentanoic acid with ethanol in the presence of a strong acid catalyst such as sulfuric acid. The reaction typically involves heating the mixture under reflux conditions to facilitate the formation of the ester.
Industrial Production Methods: In an industrial setting, the production of this compound may involve continuous flow processes to enhance efficiency and yield. The use of advanced catalytic systems and optimized reaction conditions can further improve the scalability of the synthesis.
Types of Reactions:
Oxidation: this compound can undergo oxidation reactions to form corresponding oxo derivatives.
Reduction: The compound can be reduced to form primary amines or alcohols, depending on the reducing agents used.
Substitution: Nucleophilic substitution reactions can occur at the ester or amino group, leading to the formation of various substituted derivatives.
Common Reagents and Conditions:
Oxidation: Common oxidizing agents include potassium permanganate and chromium trioxide.
Reduction: Reducing agents such as lithium aluminum hydride or sodium borohydride are typically used.
Substitution: Reagents like alkyl halides or acyl chlorides can be used for substitution reactions.
Major Products Formed:
Oxidation: Formation of oxo derivatives.
Reduction: Formation of primary amines or alcohols.
Substitution: Formation of substituted esters or amines.
Scientific Research Applications
Ethyl 2-amino-4-methylpentanoate has a wide range of applications in scientific research:
Chemistry: Used as a building block in organic synthesis for the preparation of more complex molecules.
Biology: Studied for its role in metabolic pathways and as a potential precursor for bioactive compounds.
Medicine: Investigated for its potential therapeutic properties and as a prodrug for delivering active pharmaceutical ingredients.
Industry: Utilized in the synthesis of specialty chemicals and as an intermediate in the production of various industrial products.
Mechanism of Action
The mechanism of action of ethyl 2-amino-4-methylpentanoate involves its interaction with specific molecular targets and pathways. As an ester derivative of leucine, it may participate in metabolic processes related to amino acid metabolism. The compound can be hydrolyzed to release leucine, which then exerts its effects through various biochemical pathways, including protein synthesis and energy production.
Comparison with Similar Compounds
Methyl 2-amino-4-methylpentanoate: A methyl ester derivative with similar chemical properties but different reactivity due to the presence of a methyl group instead of an ethyl group.
Ethyl 2-hydroxy-4-methylpentanoate:
Ethyl 3-amino-4-methylpentanoate: An isomer with the amino group positioned differently, leading to variations in reactivity and applications.
Uniqueness: this compound is unique due to its specific ester structure, which imparts distinct chemical properties and reactivity
Properties
IUPAC Name |
ethyl 2-amino-4-methylpentanoate |
Source
|
---|---|---|
Details | Computed by Lexichem TK 2.7.0 (PubChem release 2021.05.07) | |
Source | PubChem | |
URL | https://pubchem.ncbi.nlm.nih.gov | |
Description | Data deposited in or computed by PubChem | |
InChI |
InChI=1S/C8H17NO2/c1-4-11-8(10)7(9)5-6(2)3/h6-7H,4-5,9H2,1-3H3 |
Source
|
Details | Computed by InChI 1.0.6 (PubChem release 2021.05.07) | |
Source | PubChem | |
URL | https://pubchem.ncbi.nlm.nih.gov | |
Description | Data deposited in or computed by PubChem | |
InChI Key |
QIGLJVBIRIXQRN-UHFFFAOYSA-N |
Source
|
Details | Computed by InChI 1.0.6 (PubChem release 2021.05.07) | |
Source | PubChem | |
URL | https://pubchem.ncbi.nlm.nih.gov | |
Description | Data deposited in or computed by PubChem | |
Canonical SMILES |
CCOC(=O)C(CC(C)C)N |
Source
|
Details | Computed by OEChem 2.3.0 (PubChem release 2021.05.07) | |
Source | PubChem | |
URL | https://pubchem.ncbi.nlm.nih.gov | |
Description | Data deposited in or computed by PubChem | |
Molecular Formula |
C8H17NO2 |
Source
|
Details | Computed by PubChem 2.1 (PubChem release 2021.05.07) | |
Source | PubChem | |
URL | https://pubchem.ncbi.nlm.nih.gov | |
Description | Data deposited in or computed by PubChem | |
DSSTOX Substance ID |
DTXSID00875770 |
Source
|
Record name | Leucine, ethyl ester | |
Source | EPA DSSTox | |
URL | https://comptox.epa.gov/dashboard/DTXSID00875770 | |
Description | DSSTox provides a high quality public chemistry resource for supporting improved predictive toxicology. | |
Molecular Weight |
159.23 g/mol |
Source
|
Details | Computed by PubChem 2.1 (PubChem release 2021.05.07) | |
Source | PubChem | |
URL | https://pubchem.ncbi.nlm.nih.gov | |
Description | Data deposited in or computed by PubChem | |
CAS No. |
2743-40-0, 2743-60-4 |
Source
|
Record name | Ethyl L-leucinate hydrochloride | |
Source | ChemIDplus | |
URL | https://pubchem.ncbi.nlm.nih.gov/substance/?source=chemidplus&sourceid=0002743400 | |
Description | ChemIDplus is a free, web search system that provides access to the structure and nomenclature authority files used for the identification of chemical substances cited in National Library of Medicine (NLM) databases, including the TOXNET system. | |
Record name | Leucine, ethyl ester | |
Source | EPA DSSTox | |
URL | https://comptox.epa.gov/dashboard/DTXSID00875770 | |
Description | DSSTox provides a high quality public chemistry resource for supporting improved predictive toxicology. | |
Disclaimer and Information on In-Vitro Research Products
Please be aware that all articles and product information presented on BenchChem are intended solely for informational purposes. The products available for purchase on BenchChem are specifically designed for in-vitro studies, which are conducted outside of living organisms. In-vitro studies, derived from the Latin term "in glass," involve experiments performed in controlled laboratory settings using cells or tissues. It is important to note that these products are not categorized as medicines or drugs, and they have not received approval from the FDA for the prevention, treatment, or cure of any medical condition, ailment, or disease. We must emphasize that any form of bodily introduction of these products into humans or animals is strictly prohibited by law. It is essential to adhere to these guidelines to ensure compliance with legal and ethical standards in research and experimentation.