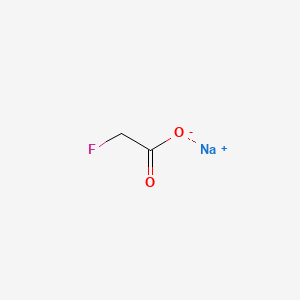
sodium fluoroacetate
Overview
Description
Sodium fluoroacetate, also known as compound 1080, is an organofluorine chemical compound with the chemical formula FCH₂CO₂Na. It is the sodium salt of fluoroacetic acid and contains sodium cations (Na⁺) and fluoroacetate anions (FCH₂CO₂⁻). This colorless salt is odorless and has a taste similar to that of table salt (sodium chloride). This compound is primarily used as a rodenticide and has been reported to be highly toxic .
Mechanism of Action
Sodium monofluoroacetate, also known as Compound 1080, is a highly toxic compound primarily used as a pesticide . It is chemically and toxicologically identical to the fluoroacetate found in some toxic plants .
Target of Action
The primary target of sodium monofluoroacetate is the tricarboxylic acid (TCA) cycle, also known as the Krebs cycle . This cycle is a crucial part of cellular metabolism, responsible for the oxidation of acetyl-CoA derived from carbohydrates, fats, and proteins to produce energy.
Mode of Action
Sodium monofluoroacetate acts by inhibiting energy production in most cells of the body . Following absorption, it undergoes intracellular conversion to produce fluorocitrate, which inhibits the TCA cycle . Fluorocitrate inhibits aconitase and the oxidation of citric acid, resulting in blockage of the TCA cycle .
Biochemical Pathways
The inhibition of aconitase interferes with cellular respiration and the metabolism of carbohydrates, fats, and proteins . This blockage of the TCA cycle leads to energy depletion, citric and lactic acid accumulation, and a decrease in blood pH . The inability to oxidize fatty acids via the TCA cycle leads to ketone body accumulation and worsening acidosis .
Pharmacokinetics
It is known that the compound is readily absorbed and distributed in the body, where it is converted to fluorocitrate .
Result of Action
The result of sodium monofluoroacetate’s action is a severe disruption of energy metabolism. This leads to a range of effects, including neurotoxicity and acute toxicity in mammals . The main organs affected are the central nervous, respiratory, and cardiovascular systems .
Action Environment
Sodium monofluoroacetate is labile in the environment, being readily degraded by different microorganisms . . For instance, the presence of fluoride ions in the environment can interact with fluoroacetate and affect its toxicity .
Biochemical Analysis
Biochemical Properties
Sodium monofluoroacetate’s extreme toxicity to mammals and insects stems from its similarity to acetate, which has a pivotal role in cellular metabolism . It is metabolized to fluorocitrate, which then interferes with adenosine triphosphate (ATP) formation in the Krebs cycle . This disruption of the tricarboxylic acid cycle results in a buildup of citrate and can cause convulsive seizures .
Cellular Effects
Sodium monofluoroacetate affects various types of cells and cellular processes. It influences cell function by disrupting energy production, leading to impaired oxidative metabolism . Energy production is reduced, and intermediates of the tricarboxylic acid cycle subsequent to citrate are depleted . This disruption can impact cell signaling pathways, gene expression, and cellular metabolism.
Molecular Mechanism
Sodium monofluoroacetate exerts its effects at the molecular level through a series of binding interactions with biomolecules. It combines with coenzyme A (CoA-SH) to form fluoroacetyl CoA, which can substitute for acetyl CoA in the tricarboxylic acid cycle and reacts with citrate synthase to produce fluorocitrate . This metabolite then binds very tightly to aconitase, thereby halting the cycle .
Temporal Effects in Laboratory Settings
The effects of sodium monofluoroacetate can change over time in laboratory settings. Clinical findings after ingestion of a sufficient amount of sodium monofluoroacetate to cause poisoning usually develop within 30 minutes to 2.5 hours of exposure . They might be delayed for as long as 20 hours .
Dosage Effects in Animal Models
The effects of sodium monofluoroacetate vary with different dosages in animal models. Acute and long-term effects of a single, relatively high oral dose (0.25 and 0.30 mg/kg) of sodium monofluoroacetate on the survival and productivity of sheep were evaluated . High doses can result in fatal poisonings .
Metabolic Pathways
Sodium monofluoroacetate is involved in the tricarboxylic acid cycle, a crucial metabolic pathway. It is metabolized to fluorocitrate, which interferes with the cycle and halts energy production .
Transport and Distribution
Sodium monofluoroacetate is transported and distributed within cells and tissues in a manner consistent with its water-soluble nature . Plasma levels of sodium monofluoroacetate were roughly double that of other organs .
Subcellular Localization
Given its role in the tricarboxylic acid cycle, it is likely to be found in the mitochondria where this cycle occurs .
Preparation Methods
Sodium fluoroacetate is synthesized by treating sodium chloroacetate with potassium fluoride. The reaction is as follows: [ \text{ClCH}_2\text{COONa} + \text{KF} \rightarrow \text{FCH}_2\text{COONa} + \text{KCl} ] This method involves the use of potassium fluoride as a fluorinating agent under mild conditions, resulting in a high fluorine conversion rate and good product quality .
Chemical Reactions Analysis
Sodium fluoroacetate undergoes several types of chemical reactions, including:
Hydrolysis: In aqueous solutions, this compound can hydrolyze to form fluoroacetic acid.
Substitution: The fluoro group can be substituted by other nucleophiles under specific conditions.
Oxidation and Reduction: this compound can participate in redox reactions, although these are less common.
Common reagents used in these reactions include water for hydrolysis and various nucleophiles for substitution reactions. The major products formed depend on the specific reaction conditions and reagents used .
Scientific Research Applications
Sodium fluoroacetate has a wide range of scientific research applications:
Chemistry: It is used as a reagent in organic synthesis and as a precursor for the synthesis of other fluorinated compounds.
Biology: this compound is used in studies related to metabolic pathways and enzyme inhibition.
Medicine: It has been explored for its potential use in treating certain metabolic disorders and as a radiolabeling agent in diagnostic imaging.
Industry: This compound is used in pest control, particularly for controlling rodent populations in agricultural settings
Comparison with Similar Compounds
Sodium fluoroacetate is unique due to its high toxicity and specific mechanism of action. Similar compounds include:
Potassium fluoroacetate: Another salt of fluoroacetic acid with similar toxic properties.
Sodium monofluoroacetate: Often used interchangeably with this compound, but with slight differences in solubility and reactivity.
Fluoroacetamide: A related compound with similar toxic effects but different chemical structure and properties
This compound stands out due to its widespread use in pest control and its specific inhibition of the citric acid cycle, making it a valuable tool in both scientific research and practical applications.
Properties
CAS No. |
62-74-8 |
---|---|
Molecular Formula |
C2H3FNaO2 |
Molecular Weight |
101.03 g/mol |
IUPAC Name |
sodium;2-fluoroacetate |
InChI |
InChI=1S/C2H3FO2.Na/c3-1-2(4)5;/h1H2,(H,4,5); |
InChI Key |
USQSMTBTMHHOKG-UHFFFAOYSA-N |
SMILES |
C(C(=O)[O-])F.[Na+] |
Canonical SMILES |
C(C(=O)O)F.[Na] |
boiling_point |
Decomposes (NIOSH, 2024) decomposes Decomposes |
Color/Form |
White solid Fluffy, colorless to white (sometimes dyed black) powder [Note: A liquid above 95 degrees F] Colorless powder Fine, white powder or monoclinic crystals |
density |
greater than 1 (temperature not listed) (USCG, 1999) >1 |
melting_point |
392 °F Decomposes at 392 °F. (EPA, 1998) 200-202 °C; decomposes above melting point 392 °F (decomposes) 392 °F |
physical_description |
Sodium fluoroacetate appears as a fine, white, odorless, powdered solid. Toxic by ingestion, inhalation and skin absorption. Used as a rodenticide. Fluffy, colorless to white (sometimes dyed black), odorless powder. [Note: A liquid above 95 degrees F.] [rodenticide] [NIOSH] COLOURLESS SOLID IN VARIOUS FORMS. Fluffy, colorless to white (sometimes dyed black), odorless powder. Fluffy, colorless to white (sometimes dyed black), odorless powder. [Note: A liquid above 95 °F.] [rodenticide] |
Related CAS |
144-49-0 (Parent) |
solubility |
Miscible (NIOSH, 2024) Solubility (g/100 g) at 25 °C in: water: 111 Almost insoluble in ethanol, acetone, and petroleum oils Solubility (g/100 g) at 25 °C in: methanol: 5; ethanol: 1.4; acetone: 0.04; carbon tetrachloride: 0.004 Practically insoluble in MeCO3 Solubility in water: good Miscible |
vapor_pressure |
0 mmHg at 68 °F (EPA, 1998) VP: Non-volatile Approx 0.0 mm Hg at 20 °C low Low |
Origin of Product |
United States |
Retrosynthesis Analysis
AI-Powered Synthesis Planning: Our tool employs the Template_relevance Pistachio, Template_relevance Bkms_metabolic, Template_relevance Pistachio_ringbreaker, Template_relevance Reaxys, Template_relevance Reaxys_biocatalysis model, leveraging a vast database of chemical reactions to predict feasible synthetic routes.
One-Step Synthesis Focus: Specifically designed for one-step synthesis, it provides concise and direct routes for your target compounds, streamlining the synthesis process.
Accurate Predictions: Utilizing the extensive PISTACHIO, BKMS_METABOLIC, PISTACHIO_RINGBREAKER, REAXYS, REAXYS_BIOCATALYSIS database, our tool offers high-accuracy predictions, reflecting the latest in chemical research and data.
Strategy Settings
Precursor scoring | Relevance Heuristic |
---|---|
Min. plausibility | 0.01 |
Model | Template_relevance |
Template Set | Pistachio/Bkms_metabolic/Pistachio_ringbreaker/Reaxys/Reaxys_biocatalysis |
Top-N result to add to graph | 6 |
Feasible Synthetic Routes
Disclaimer and Information on In-Vitro Research Products
Please be aware that all articles and product information presented on BenchChem are intended solely for informational purposes. The products available for purchase on BenchChem are specifically designed for in-vitro studies, which are conducted outside of living organisms. In-vitro studies, derived from the Latin term "in glass," involve experiments performed in controlled laboratory settings using cells or tissues. It is important to note that these products are not categorized as medicines or drugs, and they have not received approval from the FDA for the prevention, treatment, or cure of any medical condition, ailment, or disease. We must emphasize that any form of bodily introduction of these products into humans or animals is strictly prohibited by law. It is essential to adhere to these guidelines to ensure compliance with legal and ethical standards in research and experimentation.