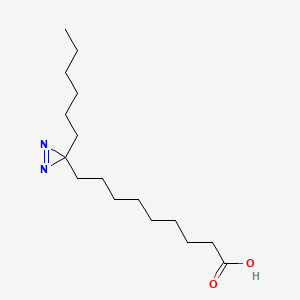
Photo-Palmitic acid
- Click on QUICK INQUIRY to receive a quote from our team of experts.
- With the quality product at a COMPETITIVE price, you can focus more on your research.
Overview
Description
Photo-Palmitic acid is a derivative of palmitic acid, a 16-carbon long-chain saturated fatty acid. Palmitic acid is one of the most common saturated fatty acids found in the human body and various dietary sources such as palm oil, butter, and meat. This compound is specifically designed for use in photo-affinity labeling, a technique used to study protein-protein interactions and other molecular interactions within cells.
Mechanism of Action
Target of Action
Palmitic acid (PA) is the most common saturated fatty acid in humans, accounting for 20–30% of total fatty acids in the human body . It mediates palmitoylation through its conversion into palmitoyl coenzyme A . The primary targets of PA are proteins that undergo palmitoylation, a process that affects many proteins . One such target is the plant homeodomain finger protein 2 (PHF2), which is palmitoylated by the zinc finger DHHC-type palmitoyltransferase 23 (ZDHHC23) . PHF2 functions as a tumor suppressor by acting as an E3 ubiquitin ligase of sterol regulatory element-binding protein 1c (SREBP1c), a master transcription factor of lipogenesis .
Mode of Action
The reversible thioester linkage of palmitic acid on cysteines, known as protein S-palmitoylation, facilitates the membrane association and proper subcellular localization of proteins . In the case of PHF2, ZDHHC23 mediates its palmitoylation, subsequently enhancing ubiquitin-dependent degradation of PHF2 . This process directly destabilizes SREBP1c and reduces SREBP1c-dependent lipogenesis .
Biochemical Pathways
Palmitic acid plays a crucial role in lipid metabolism. It acts as a molecular checkpoint of lipid reprogramming in HepG2 and Hep3B cells . The increase of free saturated fatty acids such as palmitic acid produces a metabolic inflammatory response in astrocytes generally associated with damaging mechanisms such as oxidative stress, endoplasmic reticulum stress, and autophagic defects .
Pharmacokinetics
Palmitic acid can be provided in the diet or synthesized endogenously from other fatty acids, carbohydrates, and amino acids . It represents 20–30% of total fatty acids in membrane phospholipids and adipose triacylglycerols . .
Result of Action
The palmitoylation of PHF2 enhances the ubiquitin-dependent degradation of PHF2 . This process directly destabilizes SREBP1c and reduces SREBP1c-dependent lipogenesis . Notably, SREBP1c increases free fatty acids in hepatocellular carcinoma (HCC) cells, and the consequent PA induction triggers the PHF2/SREBP1c axis .
Action Environment
The action of palmitic acid can be influenced by environmental factors such as diet and lifestyle. For instance, an excessive intake of carbohydrates, particularly mono and disaccharides, and a sedentary lifestyle can disrupt the mechanisms to maintain a steady state of PA concentration, leading to an overaccumulation of tissue PA resulting in dyslipidemia, hyperglycemia, increased ectopic fat accumulation, and increased inflammatory tone via toll-like receptor 4 . Therefore, levels of dietary PA should be carefully monitored in patients with HCC .
Biochemical Analysis
Biochemical Properties
Photo-Palmitic acid participates in several biochemical reactions, primarily involving lipid metabolism and signaling pathways. It interacts with enzymes such as fatty acid synthase and acetyl-CoA carboxylase, which are essential for fatty acid biosynthesis. Additionally, this compound is involved in protein palmitoylation, a post-translational modification where palmitic acid is covalently attached to cysteine residues on proteins, influencing their localization and function. This interaction is crucial for the proper functioning of proteins involved in signal transduction and membrane trafficking .
Cellular Effects
This compound affects various cell types and cellular processes. It influences cell signaling pathways, including the activation of toll-like receptor 4 (TLR4), which plays a role in inflammatory responses. This compound also impacts gene expression by modulating transcription factors such as nuclear factor kappa-light-chain-enhancer of activated B cells (NF-κB). Furthermore, it affects cellular metabolism by altering the balance between lipid synthesis and degradation, leading to changes in lipid droplet formation and mitochondrial function .
Molecular Mechanism
At the molecular level, this compound exerts its effects through several mechanisms. It binds to specific proteins, such as G-protein coupled receptors (GPCRs), influencing their activity and downstream signaling pathways. This compound can also inhibit or activate enzymes involved in lipid metabolism, such as lipases and acyl-CoA synthetases. Additionally, it induces changes in gene expression by interacting with transcription factors and co-regulators, leading to alterations in cellular functions and metabolic processes .
Temporal Effects in Laboratory Settings
In laboratory settings, the effects of this compound can vary over time. Its stability and degradation are influenced by factors such as temperature, pH, and exposure to light. Long-term studies have shown that prolonged exposure to this compound can lead to sustained changes in cellular function, including alterations in lipid metabolism and inflammatory responses. These effects are often studied using in vitro cell culture models and in vivo animal models to understand the temporal dynamics of this compound’s actions .
Dosage Effects in Animal Models
The effects of this compound in animal models are dose-dependent. At low doses, it can promote beneficial effects such as enhanced lipid metabolism and improved cellular function. At high doses, this compound can induce toxic effects, including lipotoxicity, inflammation, and oxidative stress. These adverse effects are often observed in organs such as the liver, heart, and kidneys, highlighting the importance of dose optimization in experimental studies .
Metabolic Pathways
This compound is involved in several metabolic pathways, including de novo lipogenesis and fatty acid oxidation. It interacts with enzymes such as acetyl-CoA carboxylase and fatty acid synthase, which are crucial for the synthesis of fatty acids. Additionally, this compound can be incorporated into complex lipids such as triglycerides and phospholipids, influencing cellular lipid composition and metabolic flux. These interactions play a significant role in maintaining cellular energy balance and membrane integrity .
Transport and Distribution
Within cells and tissues, this compound is transported and distributed through various mechanisms. It can be taken up by cells via fatty acid transporters such as CD36 and fatty acid-binding proteins (FABPs). Once inside the cell, this compound can be incorporated into lipid droplets or transported to specific organelles such as the endoplasmic reticulum and mitochondria. These processes are essential for the proper localization and function of this compound within the cell .
Subcellular Localization
The subcellular localization of this compound is critical for its activity and function. It is often found in lipid droplets, where it plays a role in lipid storage and metabolism. Additionally, this compound can localize to the plasma membrane, influencing membrane fluidity and signaling pathways. Post-translational modifications, such as palmitoylation, can direct this compound to specific compartments within the cell, such as the Golgi apparatus and endosomes, where it participates in various cellular processes .
Preparation Methods
Synthetic Routes and Reaction Conditions: The synthesis of Photo-Palmitic acid typically involves the introduction of a photo-reactive group to the palmitic acid molecule. This can be achieved through several steps:
Activation of Palmitic Acid: Palmitic acid is first activated by converting it into its acyl chloride form using thionyl chloride or oxalyl chloride.
Introduction of Photo-reactive Group: The activated palmitic acid is then reacted with a photo-reactive compound such as diazirine, benzophenone, or aryl azide under controlled conditions to form this compound.
Industrial Production Methods: Industrial production of this compound follows similar synthetic routes but on a larger scale. The process involves:
Bulk Activation: Large quantities of palmitic acid are activated using industrial-grade thionyl chloride.
Photo-reactive Group Addition: The activated palmitic acid is then reacted with photo-reactive compounds in large reactors under controlled temperature and pressure conditions to ensure high yield and purity.
Chemical Reactions Analysis
Types of Reactions: Photo-Palmitic acid undergoes several types of chemical reactions, including:
Oxidation: this compound can be oxidized to form various oxidized derivatives.
Reduction: Reduction reactions can convert this compound back to its parent palmitic acid or other reduced forms.
Substitution: The photo-reactive group in this compound can be substituted with other functional groups under specific conditions.
Common Reagents and Conditions:
Oxidation: Common oxidizing agents include potassium permanganate and chromium trioxide.
Reduction: Reducing agents such as lithium aluminum hydride or sodium borohydride are used.
Substitution: Substitution reactions often require catalysts such as palladium or platinum.
Major Products:
Oxidation Products: Oxidized derivatives of this compound.
Reduction Products: Reduced forms of this compound or palmitic acid.
Substitution Products: Various substituted derivatives depending on the substituent introduced.
Scientific Research Applications
Photo-Palmitic acid has a wide range of applications in scientific research:
Chemistry: Used in photo-affinity labeling to study molecular interactions and protein functions.
Biology: Helps in identifying protein-protein interactions and mapping protein networks within cells.
Medicine: Used in drug discovery and development to identify potential drug targets and understand drug mechanisms.
Industry: Applied in the development of new materials and chemical processes.
Comparison with Similar Compounds
Palmitic Acid: The parent compound, commonly found in dietary sources and the human body.
Palmitoleic Acid: A monounsaturated fatty acid with one double bond.
Stearic Acid: An 18-carbon saturated fatty acid.
Uniqueness of Photo-Palmitic Acid: this compound is unique due to its photo-reactive group, which allows it to be used in photo-affinity labeling. This property makes it a valuable tool in studying molecular interactions that are not easily accessible with other compounds.
Properties
IUPAC Name |
9-(3-hexyldiazirin-3-yl)nonanoic acid |
Source
|
---|---|---|
Details | Computed by Lexichem TK 2.7.0 (PubChem release 2021.10.14) | |
Source | PubChem | |
URL | https://pubchem.ncbi.nlm.nih.gov | |
Description | Data deposited in or computed by PubChem | |
InChI |
InChI=1S/C16H30N2O2/c1-2-3-4-10-13-16(17-18-16)14-11-8-6-5-7-9-12-15(19)20/h2-14H2,1H3,(H,19,20) |
Source
|
Details | Computed by InChI 1.0.6 (PubChem release 2021.10.14) | |
Source | PubChem | |
URL | https://pubchem.ncbi.nlm.nih.gov | |
Description | Data deposited in or computed by PubChem | |
InChI Key |
JIEJPTHNTCZRGW-UHFFFAOYSA-N |
Source
|
Details | Computed by InChI 1.0.6 (PubChem release 2021.10.14) | |
Source | PubChem | |
URL | https://pubchem.ncbi.nlm.nih.gov | |
Description | Data deposited in or computed by PubChem | |
Canonical SMILES |
CCCCCCC1(N=N1)CCCCCCCCC(=O)O |
Source
|
Details | Computed by OEChem 2.3.0 (PubChem release 2021.10.14) | |
Source | PubChem | |
URL | https://pubchem.ncbi.nlm.nih.gov | |
Description | Data deposited in or computed by PubChem | |
Molecular Formula |
C16H30N2O2 |
Source
|
Details | Computed by PubChem 2.2 (PubChem release 2021.10.14) | |
Source | PubChem | |
URL | https://pubchem.ncbi.nlm.nih.gov | |
Description | Data deposited in or computed by PubChem | |
Molecular Weight |
282.42 g/mol |
Source
|
Details | Computed by PubChem 2.2 (PubChem release 2021.10.14) | |
Source | PubChem | |
URL | https://pubchem.ncbi.nlm.nih.gov | |
Description | Data deposited in or computed by PubChem | |
Disclaimer and Information on In-Vitro Research Products
Please be aware that all articles and product information presented on BenchChem are intended solely for informational purposes. The products available for purchase on BenchChem are specifically designed for in-vitro studies, which are conducted outside of living organisms. In-vitro studies, derived from the Latin term "in glass," involve experiments performed in controlled laboratory settings using cells or tissues. It is important to note that these products are not categorized as medicines or drugs, and they have not received approval from the FDA for the prevention, treatment, or cure of any medical condition, ailment, or disease. We must emphasize that any form of bodily introduction of these products into humans or animals is strictly prohibited by law. It is essential to adhere to these guidelines to ensure compliance with legal and ethical standards in research and experimentation.