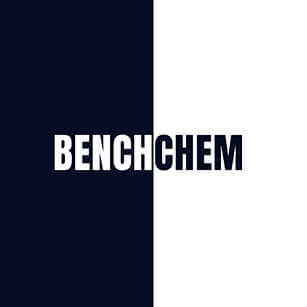
1-(1,3-oxazolidin-3-yl)ethan-1-one
- Click on QUICK INQUIRY to receive a quote from our team of experts.
- With the quality product at a COMPETITIVE price, you can focus more on your research.
Overview
Description
1-(1,3-Oxazolidin-3-yl)ethan-1-one is a heterocyclic organic compound featuring an oxazolidine ring
Preparation Methods
Synthetic Routes and Reaction Conditions
1-(1,3-Oxazolidin-3-yl)ethan-1-one can be synthesized through several methods. One common approach involves the intramolecular cyclization of N-allylcarbamates using hypervalent iodine compounds in a hexafluoroisopropanol medium . Another method includes the recyclization of 3-(2-hydroxyethyl)-1-sulfonyluracils by the action of sodium hydride at room temperature .
Industrial Production Methods
Industrial production of this compound typically involves large-scale synthesis using optimized reaction conditions to ensure high yield and purity. The specific methods and conditions may vary depending on the desired application and production scale.
Chemical Reactions Analysis
Types of Reactions
1-(1,3-Oxazolidin-3-yl)ethan-1-one undergoes various chemical reactions, including:
Oxidation: The compound can be oxidized to form different oxazolidinone derivatives.
Reduction: Reduction reactions can yield various reduced forms of the compound.
Substitution: The oxazolidine ring allows for substitution reactions, leading to the formation of diverse derivatives.
Common Reagents and Conditions
Common reagents used in these reactions include hypervalent iodine compounds for cyclization, sodium hydride for recyclization, and various oxidizing and reducing agents depending on the desired transformation .
Major Products
The major products formed from these reactions include various oxazolidinone derivatives, which can be further functionalized for specific applications.
Scientific Research Applications
1-(1,3-Oxazolidin-3-yl)ethan-1-one has a wide range of scientific research applications:
Mechanism of Action
The mechanism of action of 1-(1,3-oxazolidin-3-yl)ethan-1-one involves its interaction with specific molecular targets and pathways. For example, oxazolidinone derivatives, such as linezolid, inhibit bacterial protein synthesis by binding to the peptidyl transferase center of the bacterial ribosome . This mechanism is crucial for their antibacterial activity.
Comparison with Similar Compounds
1-(1,3-Oxazolidin-3-yl)ethan-1-one can be compared with other similar compounds, such as:
Properties
CAS No. |
3672-60-4 |
---|---|
Molecular Formula |
C5H9NO2 |
Molecular Weight |
115.13 g/mol |
IUPAC Name |
1-(1,3-oxazolidin-3-yl)ethanone |
InChI |
InChI=1S/C5H9NO2/c1-5(7)6-2-3-8-4-6/h2-4H2,1H3 |
InChI Key |
BLQLHNKKPBHCIF-UHFFFAOYSA-N |
Canonical SMILES |
CC(=O)N1CCOC1 |
Purity |
95 |
Origin of Product |
United States |
Disclaimer and Information on In-Vitro Research Products
Please be aware that all articles and product information presented on BenchChem are intended solely for informational purposes. The products available for purchase on BenchChem are specifically designed for in-vitro studies, which are conducted outside of living organisms. In-vitro studies, derived from the Latin term "in glass," involve experiments performed in controlled laboratory settings using cells or tissues. It is important to note that these products are not categorized as medicines or drugs, and they have not received approval from the FDA for the prevention, treatment, or cure of any medical condition, ailment, or disease. We must emphasize that any form of bodily introduction of these products into humans or animals is strictly prohibited by law. It is essential to adhere to these guidelines to ensure compliance with legal and ethical standards in research and experimentation.