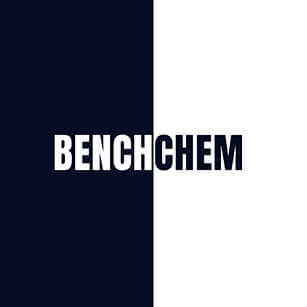
N,3-diethylaniline
- Click on QUICK INQUIRY to receive a quote from our team of experts.
- With the quality product at a COMPETITIVE price, you can focus more on your research.
Overview
Description
N,3-diethylaniline is an organic compound with the molecular formula C10H15N. It is a colorless to yellowish liquid with an aniline-like odor. This compound is used as an intermediate in the production of dyes, pharmaceuticals, and other chemicals. It is also known for its role as a reaction catalyst and a reducing agent in organic synthesis .
Mechanism of Action
Target of Action
N,3-Diethylaniline is an organic compound with the molecular formula (C2H5)2NC6H5 Similar compounds such as 3,4-dimethylaniline have been found to interact with enzymes like nicotinate-nucleotide–dimethylbenzimidazole phosphoribosyltransferase .
Mode of Action
It’s known that anilines and their derivatives generally undergo enzymatic reactions that lead to their activation and subsequent interaction with their targets .
Biochemical Pathways
Similar compounds like butachlor, a chloroacetamide herbicide, inhibit the biosynthesis of very-long-chain fatty acids (vlcfas) in microsomes
Pharmacokinetics
The compound’s molecular weight (1492328 g/mol) and structure suggest that it might be absorbed and distributed in the body
Result of Action
Anilines and their derivatives are known to have various effects on cellular processes, including the inhibition of certain enzymatic reactions .
Action Environment
Environmental factors can influence the action, efficacy, and stability of this compound. For instance, the effectiveness of biosparging to mitigate this compound in an aquifer was evaluated by measuring the time course of decrease in concentration of the compound in aerobic microcosm experiments . This suggests that the presence of oxygen and certain microbial communities can influence the degradation and hence the action of this compound.
Biochemical Analysis
Biochemical Properties
N,3-diethylaniline plays a significant role in biochemical reactions, particularly in the context of its biodegradation. It interacts with various enzymes and proteins involved in the oxidative degradation pathways. For instance, dioxygenase enzymes catalyze the initial oxidation of this compound to form catechol derivatives. These derivatives are further processed through ortho- or meta-cleavage pathways, leading to the formation of central intermediates that enter the tricarboxylic acid cycle . The interactions between this compound and these enzymes are crucial for its breakdown and subsequent assimilation into cellular metabolism.
Cellular Effects
This compound has been shown to affect various types of cells and cellular processes. In microbial cells, it can induce the expression of genes encoding for oxidative enzymes, thereby enhancing the cell’s ability to degrade aromatic compounds. This compound can also influence cell signaling pathways by modulating the activity of transcription factors involved in stress response and metabolic regulation. Additionally, this compound can impact cellular metabolism by altering the flux of metabolites through key biochemical pathways .
Molecular Mechanism
The molecular mechanism of action of this compound involves its interaction with specific biomolecules, leading to enzyme activation or inhibition. The compound binds to the active site of dioxygenase enzymes, facilitating the incorporation of molecular oxygen into the aromatic ring. This binding interaction is critical for the initial step of the oxidative degradation pathway. Furthermore, this compound can modulate gene expression by interacting with transcription factors, thereby influencing the synthesis of enzymes involved in its metabolism .
Temporal Effects in Laboratory Settings
In laboratory settings, the effects of this compound have been observed to change over time. The compound exhibits stability under aerobic conditions, allowing for its sustained degradation by microbial communities. Under anaerobic conditions, this compound is recalcitrant and shows minimal degradation. Long-term studies have demonstrated that the presence of this compound can lead to adaptive responses in microbial populations, enhancing their capacity to metabolize aromatic compounds .
Dosage Effects in Animal Models
The effects of this compound vary with different dosages in animal models. At low doses, the compound may not exhibit significant toxic effects, allowing for its metabolism and excretion. At high doses, this compound can cause adverse effects, including hepatotoxicity and nephrotoxicity. These toxic effects are likely due to the accumulation of reactive intermediates formed during its metabolism, which can damage cellular structures and impair organ function .
Metabolic Pathways
This compound is involved in several metabolic pathways, primarily those related to its oxidative degradation. The compound is initially oxidized by dioxygenase enzymes to form catechol derivatives, which are further processed through ortho- or meta-cleavage pathways. These pathways involve the action of various enzymes, including dehydrogenases and hydrolases, which facilitate the conversion of intermediates into central metabolites that enter the tricarboxylic acid cycle .
Transport and Distribution
Within cells and tissues, this compound is transported and distributed through interactions with specific transporters and binding proteins. These interactions facilitate the uptake of the compound into cells, where it can undergo metabolic processing. The localization and accumulation of this compound within cellular compartments are influenced by its chemical properties and the presence of transport proteins that mediate its movement across membranes .
Subcellular Localization
The subcellular localization of this compound is primarily within the cytoplasm, where it interacts with enzymes involved in its metabolism. The compound may also be directed to specific organelles, such as the mitochondria, where further metabolic processing occurs. Post-translational modifications and targeting signals play a role in directing this compound to these compartments, ensuring its efficient degradation and assimilation into cellular metabolism .
Preparation Methods
Synthetic Routes and Reaction Conditions
N,3-diethylaniline can be synthesized through the alkylation of aniline with ethyl halides in the presence of a base. One common method involves the reaction of aniline with diethyl sulfate or ethyl bromide under basic conditions. The reaction typically takes place in an organic solvent such as ethanol or toluene, and the temperature is maintained between 50-100°C .
Industrial Production Methods
In industrial settings, this compound is produced by the alkylation of aniline with ethyl chloride or ethyl bromide in the presence of a catalyst such as aluminum chloride. The reaction is carried out in a continuous flow reactor, and the product is purified through distillation .
Chemical Reactions Analysis
Types of Reactions
N,3-diethylaniline undergoes various chemical reactions, including:
Oxidation: It can be oxidized to form N-ethyl-N-(3-ethylphenyl)amine oxide.
Reduction: It can be reduced to form N,3-diethylcyclohexylamine.
Substitution: It can undergo electrophilic substitution reactions, such as nitration and sulfonation.
Common Reagents and Conditions
Oxidation: Common oxidizing agents include hydrogen peroxide and peracids.
Reduction: Reducing agents such as lithium aluminum hydride or sodium borohydride are used.
Substitution: Reagents like nitric acid for nitration and sulfuric acid for sulfonation are employed.
Major Products Formed
Oxidation: N-ethyl-N-(3-ethylphenyl)amine oxide.
Reduction: N,3-diethylcyclohexylamine.
Substitution: Nitro and sulfonated derivatives of this compound.
Scientific Research Applications
N,3-diethylaniline has several applications in scientific research:
Chemistry: It is used as an intermediate in the synthesis of dyes and pigments.
Biology: It is used in the study of electron transfer processes in biological systems.
Medicine: It serves as an intermediate in the synthesis of pharmaceuticals.
Industry: It is used in the production of dyes, pigments, and other chemicals.
Comparison with Similar Compounds
Similar Compounds
N,N-Diethylaniline: Similar in structure but lacks the 3-ethyl group.
N,N-Dimethylaniline: Contains methyl groups instead of ethyl groups.
N,N-Diethyl-p-toluidine: Contains a methyl group on the benzene ring.
Uniqueness
N,3-diethylaniline is unique due to the presence of the 3-ethyl group, which influences its reactivity and the types of reactions it can undergo. This structural difference makes it distinct from other similar compounds and affects its applications in various fields .
Properties
CAS No. |
101415-17-2 |
---|---|
Molecular Formula |
C10H15N |
Molecular Weight |
149.2 |
Purity |
95 |
Origin of Product |
United States |
Disclaimer and Information on In-Vitro Research Products
Please be aware that all articles and product information presented on BenchChem are intended solely for informational purposes. The products available for purchase on BenchChem are specifically designed for in-vitro studies, which are conducted outside of living organisms. In-vitro studies, derived from the Latin term "in glass," involve experiments performed in controlled laboratory settings using cells or tissues. It is important to note that these products are not categorized as medicines or drugs, and they have not received approval from the FDA for the prevention, treatment, or cure of any medical condition, ailment, or disease. We must emphasize that any form of bodily introduction of these products into humans or animals is strictly prohibited by law. It is essential to adhere to these guidelines to ensure compliance with legal and ethical standards in research and experimentation.