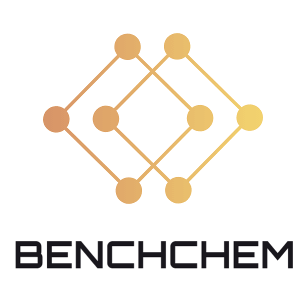
2-chloro-5-ethylbenzoic acid
- Click on QUICK INQUIRY to receive a quote from our team of experts.
- With the quality product at a COMPETITIVE price, you can focus more on your research.
Description
2-Chloro-5-ethylbenzoic acid is a chemical compound with the molecular formula C9H9ClO2 and a molecular weight of 184.62 g/mol . It is categorized as an impurity .
Synthesis Analysis
While there isn’t specific information available on the synthesis of 2-chloro-5-ethylbenzoic acid, a related compound, 5-bromo-2-chlorobenzoic acid, has been synthesized using 2-chlorobenzoic acid as a raw material . The monobromination reaction was carried out in an NBS/sulfuric acid system .Molecular Structure Analysis
The molecular structure of 2-chloro-5-ethylbenzoic acid consists of 9 carbon atoms, 9 hydrogen atoms, 1 chlorine atom, and 2 oxygen atoms .Scientific Research Applications
Occurrence, Fate, and Behavior in Aquatic Environments
Parabens, including 2-chloro-5-ethylbenzoic acid derivatives, are extensively used in various products and are known as emerging contaminants in aquatic environments. They are biodegradable but still ubiquitous in surface water and sediments due to continuous introduction into the environment. The toxicity of chlorinated parabens and their by-products, which are more stable and persistent than the parent species, needs further study to improve our understanding of their impact on health and the environment Haman, Dauchy, Rosin, & Munoz, 2015.
Analytical Methods for Determining Antioxidant Activity
The ABTS radical cation-based assay is widely used to determine antioxidant capacity. However, there are questions regarding the application of this assay due to specific reactions such as coupling, which might bias comparisons between antioxidants. Despite these concerns, ABTS-based assays are still recommended, especially for tracking changes in the same antioxidant system during storage and processing Ilyasov, Beloborodov, Selivanova, & Terekhov, 2020.
Antioxidant Capacity Reaction Pathways
Certain antioxidants can form coupling adducts with ABTS radicals, whereas others undergo oxidation without coupling. The extent of the coupling reaction's contribution to the total antioxidant capacity and the specificity and relevance of oxidation products require further elucidation Ilyasov, Beloborodov, Selivanova, & Terekhov, 2020.
properties
{ "Design of the Synthesis Pathway": "The synthesis pathway for 2-chloro-5-ethylbenzoic acid involves the chlorination of 5-ethylbenzoic acid followed by oxidation of the resulting 2-chloro-5-ethylbenzyl chloride to form the desired product.", "Starting Materials": [ "5-ethylbenzoic acid", "phosphorus pentachloride", "sodium hydroxide", "hydrogen peroxide", "water" ], "Reaction": [ "5-ethylbenzoic acid is reacted with phosphorus pentachloride to form 2-chloro-5-ethylbenzyl chloride.", "The resulting 2-chloro-5-ethylbenzyl chloride is then oxidized using a mixture of sodium hydroxide, hydrogen peroxide, and water to form 2-chloro-5-ethylbenzoic acid." ] } | |
CAS RN |
67648-08-2 |
Product Name |
2-chloro-5-ethylbenzoic acid |
Molecular Formula |
C9H9ClO2 |
Molecular Weight |
184.6 |
Purity |
95 |
Origin of Product |
United States |
Disclaimer and Information on In-Vitro Research Products
Please be aware that all articles and product information presented on BenchChem are intended solely for informational purposes. The products available for purchase on BenchChem are specifically designed for in-vitro studies, which are conducted outside of living organisms. In-vitro studies, derived from the Latin term "in glass," involve experiments performed in controlled laboratory settings using cells or tissues. It is important to note that these products are not categorized as medicines or drugs, and they have not received approval from the FDA for the prevention, treatment, or cure of any medical condition, ailment, or disease. We must emphasize that any form of bodily introduction of these products into humans or animals is strictly prohibited by law. It is essential to adhere to these guidelines to ensure compliance with legal and ethical standards in research and experimentation.