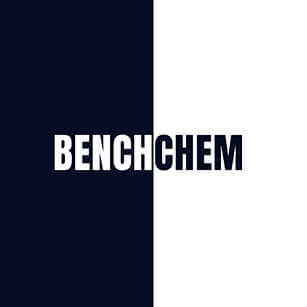
1-isocyanato-2,3-dihydro-1H-indene
Overview
Description
1-Isocyanato-2,3-dihydro-1H-indene is a bicyclic organic compound comprising a cyclopentane ring fused to a benzene ring (2,3-dihydro-1H-indene core) with an isocyanate (-NCO) functional group at position 1. The isocyanate group confers high reactivity, making this compound a versatile intermediate in organic synthesis, particularly in the formation of urethanes, polyurethanes, and pharmaceutical precursors . Its rigid bicyclic structure enhances thermal stability and modulates electronic properties, which can influence binding affinity in medicinal applications.
Preparation Methods
Synthetic Routes and Reaction Conditions
1-isocyanato-2,3-dihydro-1H-indene can be synthesized through several methods. One common approach involves the reaction of 2,3-dihydro-1H-indene with phosgene (COCl2) in the presence of a base such as triethylamine. The reaction proceeds as follows:
C9H8+COCl2→C9H7NCO+HCl
The reaction is typically carried out under anhydrous conditions to prevent the hydrolysis of phosgene. The product, this compound, is then purified by distillation or recrystallization.
Industrial Production Methods
In an industrial setting, the production of this compound may involve the use of continuous flow reactors to ensure efficient mixing and heat transfer. The use of automated systems can help control reaction parameters such as temperature, pressure, and reactant concentrations, leading to higher yields and purity of the final product.
Chemical Reactions Analysis
Types of Reactions
1-isocyanato-2,3-dihydro-1H-indene undergoes various chemical reactions, including:
Nucleophilic Addition: The isocyanate group can react with nucleophiles such as amines, alcohols, and thiols to form ureas, carbamates, and thiocarbamates, respectively.
Cycloaddition: The compound can participate in [2+2] and [4+2] cycloaddition reactions, forming cyclic adducts.
Hydrolysis: In the presence of water, the isocyanate group can hydrolyze to form the corresponding amine and carbon dioxide.
Common Reagents and Conditions
Amines: React with the isocyanate group to form ureas.
Alcohols: React with the isocyanate group to form carbamates.
Thiols: React with the isocyanate group to form thiocarbamates.
Water: Hydrolyzes the isocyanate group to form amines and carbon dioxide.
Major Products Formed
Ureas: Formed from the reaction with amines.
Carbamates: Formed from the reaction with alcohols.
Thiocarbamates: Formed from the reaction with thiols.
Amines and Carbon Dioxide: Formed from hydrolysis.
Scientific Research Applications
1-isocyanato-2,3-dihydro-1H-indene has several applications in scientific research:
Organic Synthesis: Used as a building block for the synthesis of more complex molecules.
Materials Science: Employed in the production of polymers and coatings due to its reactive isocyanate group.
Medicinal Chemistry: Investigated for its potential use in the development of pharmaceuticals, particularly as intermediates in the synthesis of biologically active compounds.
Biological Studies: Used in the study of enzyme inhibitors and other biologically relevant molecules.
Mechanism of Action
The mechanism of action of 1-isocyanato-2,3-dihydro-1H-indene primarily involves the reactivity of its isocyanate group. This group can form covalent bonds with nucleophiles, leading to the formation of various derivatives. The compound can interact with biological molecules, such as proteins and enzymes, by modifying amino groups, which can alter their function and activity.
Comparison with Similar Compounds
Comparison with Similar Compounds
The 2,3-dihydro-1H-indene scaffold is a privileged structure in medicinal chemistry due to its adaptability for diverse substitutions. Below is a comparative analysis of derivatives with varying substituents and their biological or chemical properties:
Antiproliferative Agents (Tubulin Inhibitors)
- 4,5,6-Trimethoxy-2,3-dihydro-1H-indene derivatives (e.g., compounds 12a–12r):
- Key Features : Methoxy groups at positions 4, 5, and 6 enhance electron density, improving tubulin binding.
- Activity : Compounds 12d (78.82% inhibition) and 12q (83.61% inhibition) showed potent antiproliferative effects at 0.1 mM, outperforming derivatives with electron-withdrawing groups (e.g., 12p) .
- Structural Insight : The 4,5,6-trimethoxy core is critical; introducing oxygen or carbonyl groups (e.g., 15c, 15d) significantly reduces activity .
Opioid Receptor Ligands
- Compound 4 (2,3-dihydro-1H-indene nucleus) :
Antitubercular Agents
- 4-Linked 2,3-dihydro-1H-indene derivatives (Compounds 7–13) :
Physicochemical and Functional Modifications
Sulfonamide Derivatives
- 2,3-Dihydro-1H-indene-5-sulfonamide (CAS 35203-93-1): Application: Sulfonamide groups are common in enzyme inhibitors (e.g., carbonic anhydrase).
Halogenated Derivatives
- 1-Chloro-4,7-difluoro-2,3-dihydro-1H-indene and 1-Chloro-4,6-difluoro-2,3-dihydro-1H-indene :
Unsaturated and Ketone Derivatives
- 1-Methylidene-2,3-dihydro-1H-indene (CAS 1194-56-5) :
- Indanones (e.g., compound 9): Utility: Serve as precursors for synthesizing dihydroindene derivatives (e.g., 1-phenyl-2,3-dihydro-1H-indene) via reduction or functionalization .
Comparative Data Table
Key Research Findings and Trends
Substitution-Driven Activity : Electron-donating groups (e.g., methoxy) enhance bioactivity in antiproliferative agents, while electron-withdrawing groups (e.g., halogens) improve stability for material science applications .
Core Structure Importance : Modifications to the dihydroindene core (e.g., saturation, ketones) significantly alter reactivity and biological performance, as seen in reduced tubulin inhibition with oxidized linkers .
Reactivity of Isocyanate : The -NCO group’s electrophilicity differentiates it from sulfonamides or halogens, suggesting utility in covalent drug design or polymer chemistry, though toxicity (e.g., methemoglobinemia in diarylsulfonylureas like LY186641) must be monitored .
Biological Activity
1-Isocyanato-2,3-dihydro-1H-indene is a compound of increasing interest in medicinal chemistry due to its potential biological activities. This article aims to explore its biological properties, mechanisms of action, and relevant case studies, supported by diverse scientific literature.
Chemical Structure
The chemical structure of this compound can be represented as follows:
This structure features a dihydroindene core with an isocyanate functional group, which is crucial for its biological activity.
Biological Activity Overview
Research indicates that this compound exhibits a range of biological activities, including:
- Antimicrobial Properties : Studies have shown that the compound can inhibit various bacterial strains, suggesting potential applications in treating infections.
- Anticancer Activity : Preliminary investigations indicate that this compound may induce apoptosis in cancer cell lines, making it a candidate for cancer therapy.
- Enzyme Inhibition : The compound has been reported to interact with specific enzymes, modulating their activity and influencing metabolic pathways.
The mechanism by which this compound exerts its biological effects appears to involve:
- Covalent Binding : The isocyanate group can form covalent bonds with nucleophilic sites on proteins and enzymes, altering their function.
- Receptor Modulation : Interaction with cellular receptors may lead to downstream signaling changes that affect cell proliferation and survival.
Table 1: Summary of Biological Activities
Activity Type | Observed Effects | References |
---|---|---|
Antimicrobial | Inhibition of bacterial growth | |
Anticancer | Induction of apoptosis in cancer cell lines | |
Enzyme Inhibition | Modulation of enzyme activity |
Case Study 1: Antimicrobial Activity
A recent study evaluated the antimicrobial efficacy of this compound against several pathogenic bacteria. The results indicated a significant reduction in bacterial viability at low concentrations, suggesting its potential as a broad-spectrum antimicrobial agent.
Case Study 2: Anticancer Potential
In vitro experiments demonstrated that treatment with this compound led to a marked decrease in cell viability in various cancer cell lines. Flow cytometry analysis revealed an increase in apoptotic cells, indicating that the compound may trigger programmed cell death pathways.
Q & A
Basic Research Questions
Q. What are the standard synthetic routes for 1-isocyanato-2,3-dihydro-1H-indene, and how do reaction conditions influence yield?
The synthesis typically involves introducing the isocyanato group (-NCO) into the indene scaffold via phosgenation of a primary amine precursor. A common approach includes reacting 2,3-dihydro-1H-inden-1-amine with phosgene or safer equivalents like triphosgene under anhydrous conditions. Solvent choice (e.g., dichloromethane or toluene) and temperature control (0–5°C) are critical to minimize side reactions such as urea formation . Yield optimization often requires inert atmospheres and stoichiometric precision, as highlighted in analogous indene halogenation protocols .
Q. How is this compound characterized spectroscopically?
Characterization relies on ¹H/¹³C NMR to confirm the isocyanato group’s presence (e.g., absence of NH signals and distinct carbonyl carbon shifts at ~120–130 ppm). High-resolution mass spectrometry (HRMS) validates molecular weight, while IR spectroscopy identifies the NCO stretch (~2250–2275 cm⁻¹). For example, in related indene derivatives, HRMS data with Ag⁺ adducts (e.g., [M+Ag]⁺) provide precise mass confirmation .
Advanced Research Questions
Q. How does the isocyanato group’s reactivity compare to halogen substituents (e.g., Br, Cl) in 2,3-dihydro-1H-indene derivatives?
The isocyanato group exhibits distinct electrophilic reactivity, enabling nucleophilic additions (e.g., with amines to form ureas) and polymerization. Unlike halogens, which participate in cross-coupling (e.g., Suzuki or iron-catalyzed reactions ), the -NCO group is more versatile in forming covalent bonds under mild conditions. Comparative studies of halogenated analogs (e.g., bromo- or chloro-indenes) show that electronic effects from halogens enhance oxidative stability but limit functionalization pathways .
Q. What experimental strategies mitigate decomposition or side reactions during storage and handling of this compound?
Stability challenges arise from moisture sensitivity (hydrolysis to amines/ureas) and thermal degradation. Storage under inert gas (argon) at -20°C in anhydrous solvents (e.g., THF) is recommended. Analytical monitoring via TLC or GC-MS ensures purity over time. Heat-sensitive intermediates require reaction temperatures <50°C, as seen in analogous indene derivatives prone to oxidation .
Q. How can computational modeling predict the regioselectivity of reactions involving this compound?
Density functional theory (DFT) calculations analyze electron density distributions to predict sites for nucleophilic attack. For example, the isocyanato group’s electrophilicity at the carbonyl carbon can be quantified via Fukui indices. Such models align with experimental data from indene analogs, where substituent position (e.g., para vs. meta) directs reactivity .
Q. Pharmacological and Material Science Applications
Q. What methodologies assess the biological activity of this compound derivatives?
In vitro assays (e.g., cytotoxicity via MTT or apoptosis via caspase-3 activation) screen for anticancer potential. For anti-inflammatory activity, COX-2 inhibition assays and TNF-α suppression models are employed. Structure-activity relationship (SAR) studies compare isocyanato derivatives to halogenated indenes, noting that electron-withdrawing groups like -NCO enhance target binding affinity in enzyme inhibition .
Q. How does the isocyanato group influence polymer properties in material science applications?
The -NCO group enables polyurethane synthesis via reaction with diols, producing materials with tunable rigidity and thermal stability. Rheological studies (e.g., DSC and TGA) correlate crosslinking density with mechanical performance. Compared to halogenated indenes, isocyanato-based polymers exhibit superior adhesion and chemical resistance, critical for coatings and biomedical devices .
Q. Data Analysis and Contradictions
Q. How should researchers resolve discrepancies in reported bioactivity data for indene derivatives?
Contradictions often arise from assay variability (e.g., cell line specificity) or impurities in test compounds. Meta-analyses should standardize protocols (e.g., IC₅₀ determination) and validate purity via HPLC. For example, studies on halogenated indenes show that trace solvent residues (e.g., DMSO) can artificially inflate cytotoxicity readings .
Q. What statistical approaches are optimal for analyzing substituent effects on indene derivative reactivity?
Multivariate regression models (e.g., Hammett σ values) quantify electronic effects of substituents. Principal component analysis (PCA) clusters compounds by reactivity trends, such as enhanced nucleophilic substitution rates with electron-withdrawing groups. These methods align with comparative data for bromo-, chloro-, and fluoro-indenes .
Q. Methodological Best Practices
Q. What safety protocols are essential for handling this compound?
Due to toxicity (respiratory irritant) and moisture sensitivity, use fume hoods, PPE (gloves, goggles), and moisture-free glassware. Emergency protocols for phosgene exposure (e.g., ammonia vapor detectors) are critical during synthesis. Waste disposal follows guidelines for isocyanate-containing compounds, as outlined in analogous industrial safety frameworks .
Properties
IUPAC Name |
1-isocyanato-2,3-dihydro-1H-indene | |
---|---|---|
Details | Computed by Lexichem TK 2.7.0 (PubChem release 2021.05.07) | |
Source | PubChem | |
URL | https://pubchem.ncbi.nlm.nih.gov | |
Description | Data deposited in or computed by PubChem | |
InChI |
InChI=1S/C10H9NO/c12-7-11-10-6-5-8-3-1-2-4-9(8)10/h1-4,10H,5-6H2 | |
Details | Computed by InChI 1.0.6 (PubChem release 2021.05.07) | |
Source | PubChem | |
URL | https://pubchem.ncbi.nlm.nih.gov | |
Description | Data deposited in or computed by PubChem | |
InChI Key |
XUEHOPNFOYAYTA-UHFFFAOYSA-N | |
Details | Computed by InChI 1.0.6 (PubChem release 2021.05.07) | |
Source | PubChem | |
URL | https://pubchem.ncbi.nlm.nih.gov | |
Description | Data deposited in or computed by PubChem | |
Canonical SMILES |
C1CC2=CC=CC=C2C1N=C=O | |
Details | Computed by OEChem 2.3.0 (PubChem release 2021.05.07) | |
Source | PubChem | |
URL | https://pubchem.ncbi.nlm.nih.gov | |
Description | Data deposited in or computed by PubChem | |
Molecular Formula |
C10H9NO | |
Details | Computed by PubChem 2.1 (PubChem release 2021.05.07) | |
Source | PubChem | |
URL | https://pubchem.ncbi.nlm.nih.gov | |
Description | Data deposited in or computed by PubChem | |
DSSTOX Substance ID |
DTXSID10694550 | |
Record name | 1-Isocyanato-2,3-dihydro-1H-indene | |
Source | EPA DSSTox | |
URL | https://comptox.epa.gov/dashboard/DTXSID10694550 | |
Description | DSSTox provides a high quality public chemistry resource for supporting improved predictive toxicology. | |
Molecular Weight |
159.18 g/mol | |
Details | Computed by PubChem 2.1 (PubChem release 2021.05.07) | |
Source | PubChem | |
URL | https://pubchem.ncbi.nlm.nih.gov | |
Description | Data deposited in or computed by PubChem | |
CAS No. |
26588-21-6 | |
Record name | 1-Isocyanato-2,3-dihydro-1H-indene | |
Source | EPA DSSTox | |
URL | https://comptox.epa.gov/dashboard/DTXSID10694550 | |
Description | DSSTox provides a high quality public chemistry resource for supporting improved predictive toxicology. | |
Disclaimer and Information on In-Vitro Research Products
Please be aware that all articles and product information presented on BenchChem are intended solely for informational purposes. The products available for purchase on BenchChem are specifically designed for in-vitro studies, which are conducted outside of living organisms. In-vitro studies, derived from the Latin term "in glass," involve experiments performed in controlled laboratory settings using cells or tissues. It is important to note that these products are not categorized as medicines or drugs, and they have not received approval from the FDA for the prevention, treatment, or cure of any medical condition, ailment, or disease. We must emphasize that any form of bodily introduction of these products into humans or animals is strictly prohibited by law. It is essential to adhere to these guidelines to ensure compliance with legal and ethical standards in research and experimentation.