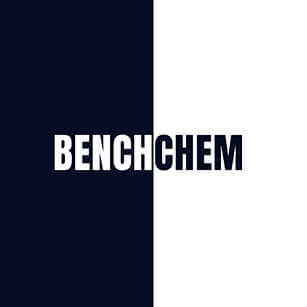
3-(2-ethylphenyl)pyrrolidine
- Click on QUICK INQUIRY to receive a quote from our team of experts.
- With the quality product at a COMPETITIVE price, you can focus more on your research.
Overview
Description
3-(2-ethylphenyl)pyrrolidine is a chemical compound that belongs to the class of synthetic cathinones.
Mechanism of Action
Target of Action
Pyrrolidine derivatives have been widely used by medicinal chemists to obtain compounds for the treatment of human diseases . The pyrrolidine ring is a common feature in many biologically active compounds .
Mode of Action
The pyrrolidine ring and its derivatives have been reported to interact with their targets in a way that contributes to the stereochemistry of the molecule . The different stereoisomers and the spatial orientation of substituents can lead to a different biological profile of drug candidates, due to the different binding mode to enantioselective proteins .
Biochemical Pathways
Pyrrolidine alkaloids have been shown to possess several important biological activities, including antioxidant, anti-inflammatory, antibacterial, antifungal, antiparasitic and anthelmintic, anticancer, anti-hyperglycemic, organ protective, and neuropharmacological activities .
Pharmacokinetics
The physicochemical parameters of pyrrolidine and its derivatives have been compared with the parent aromatic pyrrole and cyclopentane .
Result of Action
Pyrrolidine alkaloids have been shown to possess several important biological activities .
Action Environment
The influence of steric factors on the biological activity of pyrrolidine and its derivatives has been investigated .
Preparation Methods
The synthesis of 3-(2-ethylphenyl)pyrrolidine can be achieved through several methodsThis can be done through various types of cross-coupling reactions that combine heteroaromatic reagents with pyrrolidine derivatives containing the necessary substituents . Another method involves the assembly of the pyrrolidine ring containing hetaryl substituents from appropriately substituted acyclic precursors . Industrial production methods often utilize these synthetic routes to produce the compound in large quantities.
Chemical Reactions Analysis
3-(2-ethylphenyl)pyrrolidine undergoes various chemical reactions, including oxidation, reduction, and substitution. For example, the oxidation of pyrrolidine derivatives can lead to the formation of pyrrolidin-2-ones . Common reagents used in these reactions include oxidizing agents such as potassium permanganate and reducing agents like lithium aluminum hydride. The major products formed from these reactions depend on the specific conditions and reagents used.
Scientific Research Applications
3-(2-ethylphenyl)pyrrolidine has several scientific research applications. In chemistry, it is used as a building block for the synthesis of more complex molecules . In biology and medicine, it has been studied for its potential therapeutic effects, including its use as a stimulant and its impact on various biological pathways. Additionally, it has applications in the industry, particularly in the development of new materials and chemical processes .
Comparison with Similar Compounds
3-(2-ethylphenyl)pyrrolidine can be compared with other similar compounds, such as pyrrolidine alkaloids and their derivatives. These compounds share a similar five-membered nitrogen-containing ring structure but differ in their substituents and biological activities . For example, pyrrolidine-2,5-dione is another compound with a similar structure but different biological properties . The uniqueness of this compound lies in its specific substituents and their impact on its chemical and biological properties.
Similar Compounds
- Pyrrolidine-2,5-dione
- Pyrrolidine alkaloids
- Pyrrolopyrazine derivatives
- Pyrrolidinone derivatives
Biological Activity
3-(2-Ethylphenyl)pyrrolidine is a compound belonging to the pyrrolidine family, characterized by a five-membered nitrogen-containing ring. Its unique structure, featuring an ethyl group attached to a phenyl ring, contributes to its diverse biological activities and potential therapeutic applications. This article explores the biological activity of this compound, including its pharmacological effects, mechanisms of action, and relevant case studies.
- Molecular Formula : C13H17N
- Molecular Weight : Approximately 175.3 g/mol
The presence of the ethyl group at the second position of the pyrrolidine structure enhances its lipophilicity, which may influence its interaction with biological targets.
Biological Activity Overview
This compound exhibits notable biological activity, particularly in medicinal chemistry. Pyrrolidine derivatives have been studied for their potential roles as therapeutic agents due to their diverse pharmacological effects. The following sections detail specific activities observed in research.
Pharmacological Effects
- Antimicrobial Activity : Research indicates that pyrrolidine derivatives can inhibit bacterial growth, particularly against multidrug-resistant strains such as Pseudomonas aeruginosa .
- Anti-inflammatory Properties : Compounds similar to this compound have shown potential in modulating inflammatory pathways, particularly through the inhibition of the NF-κB signaling pathway .
- Cytotoxic Effects : Some studies suggest that certain pyrrolidine derivatives can induce cytotoxicity in cancer cells, indicating their potential as anticancer agents .
The biological activity of this compound is believed to stem from its ability to interact with various molecular targets:
- Enzyme Inhibition : The compound may bind to active sites on enzymes, disrupting their function and leading to therapeutic effects.
- Receptor Modulation : It can interact with specific receptors involved in signaling pathways, influencing cellular responses and potentially leading to anti-inflammatory effects.
Case Studies and Research Findings
Several studies have provided insights into the biological activity of pyrrolidine derivatives:
Table 1: Summary of Biological Activities
Synthesis and Applications
The synthesis of this compound can be achieved through various organic reactions, providing flexibility for research applications. Its versatility makes it a valuable compound in drug discovery and development.
Synthetic Routes:
- One-Pot Reactions : Efficient methods involving multiple reactants have been optimized for higher yields.
- Functionalization Strategies : Various functional groups can be introduced to modify the compound's properties and enhance its biological activity.
Properties
CAS No. |
1247925-28-5 |
---|---|
Molecular Formula |
C12H17N |
Molecular Weight |
175.3 |
Purity |
95 |
Origin of Product |
United States |
Disclaimer and Information on In-Vitro Research Products
Please be aware that all articles and product information presented on BenchChem are intended solely for informational purposes. The products available for purchase on BenchChem are specifically designed for in-vitro studies, which are conducted outside of living organisms. In-vitro studies, derived from the Latin term "in glass," involve experiments performed in controlled laboratory settings using cells or tissues. It is important to note that these products are not categorized as medicines or drugs, and they have not received approval from the FDA for the prevention, treatment, or cure of any medical condition, ailment, or disease. We must emphasize that any form of bodily introduction of these products into humans or animals is strictly prohibited by law. It is essential to adhere to these guidelines to ensure compliance with legal and ethical standards in research and experimentation.