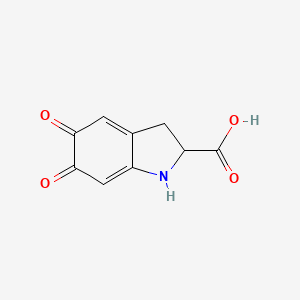
Dopachrome
Overview
Description
Dopachrome is a chemical compound that plays a crucial role in the biosynthesis of melanin, the pigment responsible for the color of skin, hair, and eyes in humans and other organisms. It is an intermediate product formed during the oxidation of L-DOPA (L-3,4-dihydroxyphenylalanine) and can further tautomerize to form 5,6-dihydroxyindole-2-carboxylic acid (DHICA) or 5,6-dihydroxyindole (DHI) .
Mechanism of Action
Target of Action
Dopachrome primarily targets the enzyme This compound Tautomerase (DCT) , also known as Tyrosinase-related protein-2 (TRP2) . DCT is a member of the Tyrosinase-related protein family and plays a crucial role in the distal steps of melanogenesis .
Mode of Action
This compound interacts with its target, DCT, through a process known as This compound conversion . In this conversion, 5,6-dihydroxyindole (DHI) is spontaneously formed through the elimination of the carboxyl group, namely decarboxylation . The non-decarboxylated product 5,6-dihydroxyindole-2-carboxylic acid (DHICA) is also formed in the presence of several factors such as DCT and Cu (II) ion as well as strongly alkaline pH .
Biochemical Pathways
This compound is an intermediate in the biosynthesis of melanin . The conversion of this compound into DHI or DHICA is a key reaction determining the amount of carboxyl groups in eumelanin . This conversion directly determines the properties of the eumelanin produced . The preference of this compound conversion mainly depends on pH, metal ions, and the enzyme DCT .
Pharmacokinetics
It’s known that the pharmacokinetics of l-dopa, a precursor to this compound, can vary greatly between individuals, and factors such as sex, age, and body weight can affect its pharmacokinetics .
Result of Action
The result of this compound’s action is the production of eumelanin , a type of melanin . This antioxidant effect is likely to be derived from the DHICA unit contained in eumelanin .
Biochemical Analysis
Biochemical Properties
Dopachrome plays a crucial role in the melanin synthesis pathway. It is produced by the diphenol oxidase activity of tyrosinase . The presence of this compound is confirmed spectrophotometrically at 475 nm . It interacts with enzymes like TYRP2 and TYRP1, leading to the production of 5,6-dihydroxyindole-2-carboxylic acid (DHICA) and 5,6-indolequinone-2-carboxylic acid (IQCA) .
Cellular Effects
This compound influences cell function by participating in the melanogenesis pathway, which produces melanin pigment. This pigment is responsible for the coloration of skin, hair, and eyes in animals . Disruptions in this pathway can lead to conditions like oculocutaneous albinism (OCA), which is linked to protein stability and enzyme activity .
Molecular Mechanism
The molecular mechanism of this compound involves its conversion to DHICA, a process catalyzed by TYRP2 . In the TYRP1-driven oxidation of DHICA, the formation of IQCA is confirmed . These reactions are part of the melanogenic pathway based on intra-melanosomal domains .
Temporal Effects in Laboratory Settings
In laboratory settings, the diphenol oxidase activity of tyrosinase achieved the maximum production of native this compound at 10 min of incubation at 37 °C . This indicates the temporal stability of this compound in these conditions.
Metabolic Pathways
This compound is involved in the melanin synthesis pathway, a metabolic pathway that produces melanin pigment. This pathway involves interactions with enzymes like TYR, TYRP2, and TYRP1 .
Subcellular Localization
The subcellular localization of this compound is likely within melanosomes, where the melanin synthesis pathway occurs
Preparation Methods
Synthetic Routes and Reaction Conditions: Dopachrome can be synthesized through the oxidation of L-DOPA. The most common method involves the use of tyrosinase, a copper-containing enzyme that catalyzes the hydroxylation of L-DOPA to dopaquinone, which then cyclizes to form this compound . The reaction is typically carried out at a pH of around 6.8 to 7.0 and at room temperature .
Industrial Production Methods: Industrial production of this compound is not widely practiced due to its instability and rapid conversion to other compounds. in laboratory settings, this compound is often prepared fresh from L-DOPA using tyrosinase or other oxidizing agents such as silver oxide .
Chemical Reactions Analysis
Types of Reactions: Dopachrome primarily undergoes decarboxylation and tautomerization reactions. The decarboxylation of this compound leads to the formation of 5,6-dihydroxyindole (DHI), while tautomerization results in 5,6-dihydroxyindole-2-carboxylic acid (DHICA) .
Common Reagents and Conditions:
Decarboxylation: This reaction is facilitated by the presence of this compound tautomerase (DCT) and metal ions such as copper (II) ions.
Tautomerization: This process can occur spontaneously or be catalyzed by enzymes such as this compound tautomerase.
Major Products:
5,6-Dihydroxyindole (DHI): Formed through decarboxylation.
5,6-Dihydroxyindole-2-carboxylic acid (DHICA): Formed through tautomerization.
Scientific Research Applications
Dopachrome has several applications in scientific research, particularly in the fields of chemistry, biology, and medicine:
Melanin Biosynthesis Studies: this compound is a key intermediate in the study of melanin biosynthesis, helping researchers understand the pathways and mechanisms involved in pigment formation.
Antioxidant Research: The conversion products of this compound, such as DHICA, have been studied for their antioxidant properties, which are important in protecting cells from oxidative stress.
Medical Research: this compound and its derivatives are investigated for their potential roles in treating conditions related to melanin production, such as vitiligo and melanoma.
Biological Studies: this compound tautomerase enzymes are studied for their evolutionary significance and roles in various organisms.
Comparison with Similar Compounds
Dopachrome is unique in its role as an intermediate in melanin biosynthesis. Similar compounds include:
L-DOPA: The precursor to this compound, involved in the initial stages of melanin synthesis.
Dopaquinone: The immediate precursor to this compound, formed through the oxidation of L-DOPA.
5,6-Dihydroxyindole (DHI): A product of this compound decarboxylation.
5,6-Dihydroxyindole-2-carboxylic acid (DHICA): A product of this compound tautomerization
This compound is distinct due to its ability to undergo both decarboxylation and tautomerization, leading to the formation of two different products that contribute to the properties of melanin .
Properties
IUPAC Name |
5,6-dioxo-2,3-dihydro-1H-indole-2-carboxylic acid | |
---|---|---|
Source | PubChem | |
URL | https://pubchem.ncbi.nlm.nih.gov | |
Description | Data deposited in or computed by PubChem | |
InChI |
InChI=1S/C9H7NO4/c11-7-2-4-1-6(9(13)14)10-5(4)3-8(7)12/h2-3,6,10H,1H2,(H,13,14) | |
Source | PubChem | |
URL | https://pubchem.ncbi.nlm.nih.gov | |
Description | Data deposited in or computed by PubChem | |
InChI Key |
VJNCICVKUHKIIV-UHFFFAOYSA-N | |
Source | PubChem | |
URL | https://pubchem.ncbi.nlm.nih.gov | |
Description | Data deposited in or computed by PubChem | |
Canonical SMILES |
C1C(NC2=CC(=O)C(=O)C=C21)C(=O)O | |
Source | PubChem | |
URL | https://pubchem.ncbi.nlm.nih.gov | |
Description | Data deposited in or computed by PubChem | |
Molecular Formula |
C9H7NO4 | |
Source | PubChem | |
URL | https://pubchem.ncbi.nlm.nih.gov | |
Description | Data deposited in or computed by PubChem | |
DSSTOX Substance ID |
DTXSID30897147, DTXSID101205460 | |
Record name | Dopachrome | |
Source | EPA DSSTox | |
URL | https://comptox.epa.gov/dashboard/DTXSID30897147 | |
Description | DSSTox provides a high quality public chemistry resource for supporting improved predictive toxicology. | |
Record name | 3,5-Dihydro-6-hydroxy-5-oxo-2H-indole-2-carboxylic acid | |
Source | EPA DSSTox | |
URL | https://comptox.epa.gov/dashboard/DTXSID101205460 | |
Description | DSSTox provides a high quality public chemistry resource for supporting improved predictive toxicology. | |
Molecular Weight |
193.16 g/mol | |
Source | PubChem | |
URL | https://pubchem.ncbi.nlm.nih.gov | |
Description | Data deposited in or computed by PubChem | |
CAS No. |
3571-34-4, 13520-94-0 | |
Record name | Dopachrome | |
Source | CAS Common Chemistry | |
URL | https://commonchemistry.cas.org/detail?cas_rn=3571-34-4 | |
Description | CAS Common Chemistry is an open community resource for accessing chemical information. Nearly 500,000 chemical substances from CAS REGISTRY cover areas of community interest, including common and frequently regulated chemicals, and those relevant to high school and undergraduate chemistry classes. This chemical information, curated by our expert scientists, is provided in alignment with our mission as a division of the American Chemical Society. | |
Explanation | The data from CAS Common Chemistry is provided under a CC-BY-NC 4.0 license, unless otherwise stated. | |
Record name | Dopachrome | |
Source | ChemIDplus | |
URL | https://pubchem.ncbi.nlm.nih.gov/substance/?source=chemidplus&sourceid=0003571344 | |
Description | ChemIDplus is a free, web search system that provides access to the structure and nomenclature authority files used for the identification of chemical substances cited in National Library of Medicine (NLM) databases, including the TOXNET system. | |
Record name | Dopachrome | |
Source | EPA DSSTox | |
URL | https://comptox.epa.gov/dashboard/DTXSID30897147 | |
Description | DSSTox provides a high quality public chemistry resource for supporting improved predictive toxicology. | |
Record name | 3,5-Dihydro-6-hydroxy-5-oxo-2H-indole-2-carboxylic acid | |
Source | EPA DSSTox | |
URL | https://comptox.epa.gov/dashboard/DTXSID101205460 | |
Description | DSSTox provides a high quality public chemistry resource for supporting improved predictive toxicology. | |
Record name | DOPACHROME | |
Source | FDA Global Substance Registration System (GSRS) | |
URL | https://gsrs.ncats.nih.gov/ginas/app/beta/substances/DWA58A6ZW7 | |
Description | The FDA Global Substance Registration System (GSRS) enables the efficient and accurate exchange of information on what substances are in regulated products. Instead of relying on names, which vary across regulatory domains, countries, and regions, the GSRS knowledge base makes it possible for substances to be defined by standardized, scientific descriptions. | |
Explanation | Unless otherwise noted, the contents of the FDA website (www.fda.gov), both text and graphics, are not copyrighted. They are in the public domain and may be republished, reprinted and otherwise used freely by anyone without the need to obtain permission from FDA. Credit to the U.S. Food and Drug Administration as the source is appreciated but not required. | |
Synthesis routes and methods I
Procedure details
Synthesis routes and methods II
Procedure details
Disclaimer and Information on In-Vitro Research Products
Please be aware that all articles and product information presented on BenchChem are intended solely for informational purposes. The products available for purchase on BenchChem are specifically designed for in-vitro studies, which are conducted outside of living organisms. In-vitro studies, derived from the Latin term "in glass," involve experiments performed in controlled laboratory settings using cells or tissues. It is important to note that these products are not categorized as medicines or drugs, and they have not received approval from the FDA for the prevention, treatment, or cure of any medical condition, ailment, or disease. We must emphasize that any form of bodily introduction of these products into humans or animals is strictly prohibited by law. It is essential to adhere to these guidelines to ensure compliance with legal and ethical standards in research and experimentation.