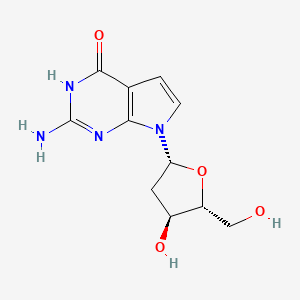
7-Deaza-2'-deoxyguanosine
Overview
Description
7-Deaza-2’-deoxyguanosine: is a modified nucleoside analog where the nitrogen atom at the seventh position of the guanine base is replaced by a carbon atom. This modification results in a compound that retains the ability to form stable Watson-Crick base pairs with cytosine, similar to canonical guanine. It is widely used in various scientific research fields due to its unique properties and applications.
Mechanism of Action
Target of Action
The primary target of 7-Deaza-2’-deoxyguanosine is CpG islands . CpG islands are GC-rich sequences found in the promoters of many genes in higher eukaryotes . They contain a high frequency of CG dinucleotides, which are substrates for DNA methylases .
Mode of Action
7-Deaza-2’-deoxyguanosine interacts with its targets by competing with the natural substrate dGTP and blocking the telomerase activity . This nucleotide, once incorporated into the telomere, alters the secondary structure of the telomere, making it difficult for telomerase to identify it for further telomere synthesis .
Biochemical Pathways
7-Deaza-2’-deoxyguanosine affects the queuosine tRNA modification pathway and the dpd gene cluster . The dpd gene cluster encodes proteins that comprise the elaborate Dpd restriction–modification system present in diverse bacteria . The dpdA, dpdB, and dpdC genes encode proteins necessary for DNA modification, with dpdD–dpdK contributing to the restriction phenotype .
Pharmacokinetics
It is known that the compound improves pcr (polymerase chain reaction) yields . This suggests that it may have good bioavailability and can effectively interact with its targets.
Biochemical Analysis
Biochemical Properties
7-Deaza-2’-deoxyguanosine interacts with various enzymes, proteins, and other biomolecules. It competes with the natural substrate dGTP and blocks the telomerase activity . The nature of these interactions involves altering the secondary structure of the telomere, making it difficult for telomerase to identify it for further telomere synthesis .
Cellular Effects
The effects of 7-Deaza-2’-deoxyguanosine on various types of cells and cellular processes are profound. It influences cell function by impacting cell signaling pathways, gene expression, and cellular metabolism .
Molecular Mechanism
At the molecular level, 7-Deaza-2’-deoxyguanosine exerts its effects through binding interactions with biomolecules, enzyme inhibition or activation, and changes in gene expression . Once incorporated into the telomere, it alters the secondary structure of the telomere .
Temporal Effects in Laboratory Settings
Over time in laboratory settings, the effects of 7-Deaza-2’-deoxyguanosine may change. It is crucial to consider the product’s stability, degradation, and any long-term effects on cellular function observed in in vitro or in vivo studies .
Metabolic Pathways
7-Deaza-2’-deoxyguanosine is involved in various metabolic pathways. It interacts with enzymes or cofactors, and it could also affect metabolic flux or metabolite levels .
Preparation Methods
Synthetic Routes and Reaction Conditions: The synthesis of 7-Deaza-2’-deoxyguanosine typically involves the selective halogenation of the nucleobase followed by the introduction of various substituents through cross-coupling reactions. For instance, regioselective iodination protocols have been developed to afford the 7- and 8-iodo isomers of 7-Deaza-2’-deoxyguanosine. These iodinated intermediates can then undergo Suzuki-Miyaura cross-coupling or click reactions to introduce aromatic side chains .
Industrial Production Methods: Industrial production of 7-Deaza-2’-deoxyguanosine often involves large-scale synthesis using similar halogenation and cross-coupling techniques. The compound is typically supplied as a lithium salt solution, which is determined by measurements of absorbance at specific wavelengths .
Chemical Reactions Analysis
Types of Reactions: 7-Deaza-2’-deoxyguanosine undergoes various chemical reactions, including:
Oxidation: The compound can be oxidized under specific conditions to form different oxidation products.
Reduction: Reduction reactions can modify the nucleobase, leading to different derivatives.
Substitution: The compound can undergo substitution reactions, such as halogenation, to introduce different functional groups.
Common Reagents and Conditions: Common reagents used in these reactions include halogenating agents for iodination, palladium catalysts for cross-coupling reactions, and various solvents like dichloromethane and acetonitrile .
Major Products: The major products formed from these reactions include iodinated derivatives, cross-coupled products with aromatic side chains, and other modified nucleosides .
Scientific Research Applications
Chemistry: In chemistry, 7-Deaza-2’-deoxyguanosine is used as a building block for the synthesis of modified oligonucleotides. These modified oligonucleotides are employed in various applications, including DNA sequencing and molecular probes .
Biology: In biological research, the compound is used to study DNA-protein interactions and the stability of DNA duplexes. It is also utilized in the synthesis of modified DNA and RNA for various experimental purposes .
Medicine: In medicine, 7-Deaza-2’-deoxyguanosine is investigated for its potential therapeutic applications, including its use as an inhibitor of viral enzymes and its role in anticancer research .
Industry: In the industrial sector, the compound is used in the production of diagnostic kits and as a reagent in various biochemical assays .
Comparison with Similar Compounds
7-Deaza-2’-deoxyadenosine: Another modified nucleoside with similar properties and applications.
2’-Deoxyguanosine: The canonical nucleoside that 7-Deaza-2’-deoxyguanosine is derived from.
7-Cyano-7-deazaguanosine: A derivative with a cyano group at the seventh position.
Uniqueness: 7-Deaza-2’-deoxyguanosine is unique due to its ability to form stable base pairs while altering the electronic properties of the nucleobase. This makes it a valuable tool in various research applications, particularly in the study of nucleic acid structure and function .
Properties
IUPAC Name |
2-amino-7-[(2R,4S,5R)-4-hydroxy-5-(hydroxymethyl)oxolan-2-yl]-3H-pyrrolo[2,3-d]pyrimidin-4-one | |
---|---|---|
Source | PubChem | |
URL | https://pubchem.ncbi.nlm.nih.gov | |
Description | Data deposited in or computed by PubChem | |
InChI |
InChI=1S/C11H14N4O4/c12-11-13-9-5(10(18)14-11)1-2-15(9)8-3-6(17)7(4-16)19-8/h1-2,6-8,16-17H,3-4H2,(H3,12,13,14,18)/t6-,7+,8+/m0/s1 | |
Source | PubChem | |
URL | https://pubchem.ncbi.nlm.nih.gov | |
Description | Data deposited in or computed by PubChem | |
InChI Key |
PFCLMNDDPTZJHQ-XLPZGREQSA-N | |
Source | PubChem | |
URL | https://pubchem.ncbi.nlm.nih.gov | |
Description | Data deposited in or computed by PubChem | |
Canonical SMILES |
C1C(C(OC1N2C=CC3=C2N=C(NC3=O)N)CO)O | |
Source | PubChem | |
URL | https://pubchem.ncbi.nlm.nih.gov | |
Description | Data deposited in or computed by PubChem | |
Isomeric SMILES |
C1[C@@H]([C@H](O[C@H]1N2C=CC3=C2N=C(NC3=O)N)CO)O | |
Source | PubChem | |
URL | https://pubchem.ncbi.nlm.nih.gov | |
Description | Data deposited in or computed by PubChem | |
Molecular Formula |
C11H14N4O4 | |
Source | PubChem | |
URL | https://pubchem.ncbi.nlm.nih.gov | |
Description | Data deposited in or computed by PubChem | |
DSSTOX Substance ID |
DTXSID501006781 | |
Record name | 7-(2-Deoxypentofuranosyl)-2-imino-2,7-dihydro-1H-pyrrolo[2,3-d]pyrimidin-4-ol | |
Source | EPA DSSTox | |
URL | https://comptox.epa.gov/dashboard/DTXSID501006781 | |
Description | DSSTox provides a high quality public chemistry resource for supporting improved predictive toxicology. | |
Molecular Weight |
266.25 g/mol | |
Source | PubChem | |
URL | https://pubchem.ncbi.nlm.nih.gov | |
Description | Data deposited in or computed by PubChem | |
CAS No. |
86392-75-8 | |
Record name | 7-Deaza-2′-deoxyguanosine | |
Source | CAS Common Chemistry | |
URL | https://commonchemistry.cas.org/detail?cas_rn=86392-75-8 | |
Description | CAS Common Chemistry is an open community resource for accessing chemical information. Nearly 500,000 chemical substances from CAS REGISTRY cover areas of community interest, including common and frequently regulated chemicals, and those relevant to high school and undergraduate chemistry classes. This chemical information, curated by our expert scientists, is provided in alignment with our mission as a division of the American Chemical Society. | |
Explanation | The data from CAS Common Chemistry is provided under a CC-BY-NC 4.0 license, unless otherwise stated. | |
Record name | 7-Deaza-2'-deoxyguanosine | |
Source | ChemIDplus | |
URL | https://pubchem.ncbi.nlm.nih.gov/substance/?source=chemidplus&sourceid=0086392758 | |
Description | ChemIDplus is a free, web search system that provides access to the structure and nomenclature authority files used for the identification of chemical substances cited in National Library of Medicine (NLM) databases, including the TOXNET system. | |
Record name | 7-(2-Deoxypentofuranosyl)-2-imino-2,7-dihydro-1H-pyrrolo[2,3-d]pyrimidin-4-ol | |
Source | EPA DSSTox | |
URL | https://comptox.epa.gov/dashboard/DTXSID501006781 | |
Description | DSSTox provides a high quality public chemistry resource for supporting improved predictive toxicology. | |
Record name | 7-DEAZA-2'-DEOXYGUANOSINE | |
Source | FDA Global Substance Registration System (GSRS) | |
URL | https://gsrs.ncats.nih.gov/ginas/app/beta/substances/7668FG8W4U | |
Description | The FDA Global Substance Registration System (GSRS) enables the efficient and accurate exchange of information on what substances are in regulated products. Instead of relying on names, which vary across regulatory domains, countries, and regions, the GSRS knowledge base makes it possible for substances to be defined by standardized, scientific descriptions. | |
Explanation | Unless otherwise noted, the contents of the FDA website (www.fda.gov), both text and graphics, are not copyrighted. They are in the public domain and may be republished, reprinted and otherwise used freely by anyone without the need to obtain permission from FDA. Credit to the U.S. Food and Drug Administration as the source is appreciated but not required. | |
Retrosynthesis Analysis
AI-Powered Synthesis Planning: Our tool employs the Template_relevance Pistachio, Template_relevance Bkms_metabolic, Template_relevance Pistachio_ringbreaker, Template_relevance Reaxys, Template_relevance Reaxys_biocatalysis model, leveraging a vast database of chemical reactions to predict feasible synthetic routes.
One-Step Synthesis Focus: Specifically designed for one-step synthesis, it provides concise and direct routes for your target compounds, streamlining the synthesis process.
Accurate Predictions: Utilizing the extensive PISTACHIO, BKMS_METABOLIC, PISTACHIO_RINGBREAKER, REAXYS, REAXYS_BIOCATALYSIS database, our tool offers high-accuracy predictions, reflecting the latest in chemical research and data.
Strategy Settings
Precursor scoring | Relevance Heuristic |
---|---|
Min. plausibility | 0.01 |
Model | Template_relevance |
Template Set | Pistachio/Bkms_metabolic/Pistachio_ringbreaker/Reaxys/Reaxys_biocatalysis |
Top-N result to add to graph | 6 |
Feasible Synthetic Routes
Q1: How does the introduction of substituents at the 7-position of the c7dG nucleobase impact duplex stability?
A2: Research shows that the introduction of various substituents at the 7-position of c7dG can significantly affect the stability of the resulting DNA duplex. For example, while the incorporation of 7-deaza-2'-deoxyguanosine generally destabilizes DNA duplexes, the addition of a 7-methyl group to this structure, creating 7-deaza-2′-deoxy-7-methylguanosine, increases duplex stability compared to unmodified 7-deaza-2′-deoxyguanosine. [] In another study, 7-propynyl-7-deaza-2'-deoxyguanosine demonstrated enhanced duplex stability attributed to the increased polarizability and hydrophobicity of the propynyl group. []
Q2: Can the 7-position of c7dG be further functionalized for applications like DNA labeling?
A3: Yes, the 7-position of c7dG serves as a versatile platform for incorporating various functional groups. Studies have successfully introduced aminoalkynyl groups at this position, allowing for post-synthetic modifications like attaching fluorescent labels or other moieties. [] This functionalization strategy is valuable for applications such as DNA labeling and bioconjugation.
Q3: Does the introduction of a propynyl group at the 7-position of 8-aza-7-deaza-2'-deoxyguanosine (3b) lead to a more stable duplex compared to its bromo counterpart (4c)?
A4: Yes, studies have shown that the propynyl derivative (4b) forms a more stable duplex than the bromo nucleoside (4c). This enhanced stability is attributed to the increased polarizability of the nucleobase and the hydrophobic nature of the propynyl group. []
Q4: How does the size and position of clickable residues on purine-purine base pairs influence molecular recognition?
A5: Research on purine-purine base pairs, specifically those containing isoguanosine and 8-aza-7-deazaisoguanosine, demonstrates that the size and position of clickable residues are crucial for molecular recognition. Side chains attached to the 7-position of 8-aza-7-deazaisoguanine, when paired with guanine or 5-aza-7-deazaguanine, are well-accommodated within the DNA structure. In contrast, functionalizing the 8-position of isoguanine with similar residues destabilizes the DNA. This difference highlights the importance of considering steric effects and potential interactions within the DNA groove when designing modified base pairs. []
Q5: How does the presence of c7dG in DNA affect its stability under PCR conditions?
A6: Incorporating c7dG into DNA, particularly in GC-rich regions prone to forming secondary structures, can improve the efficiency and specificity of PCR amplification. [, ] This is because the modified base disrupts the formation of stable secondary structures that can interfere with polymerase activity.
Q6: Does c7dG influence the stability of DNA towards enzymatic degradation?
A7: Studies show that incorporating c7dG can enhance the resistance of DNA to enzymatic degradation. This protection is attributed to the alteration of the DNA major groove caused by the 7-deazapurine base, disrupting the recognition or binding of certain endonucleases. []
Q7: Can c7dG be used to modulate the activity of enzymes that interact with DNA?
A8: Yes, the incorporation of c7dG into DNA can significantly impact the activity of enzymes that interact with DNA. For example, studies have shown that the presence of c7dG within the recognition sequence of certain restriction endonucleases can inhibit their activity, highlighting its potential as a tool for studying and manipulating DNA-protein interactions. [, ]
Q8: How does the incorporation of c7dG affect the ability of oligonucleotides to form triple helices?
A9: Research indicates that the substitution of this compound for guanine in oligonucleotides designed to form triple helices can significantly decrease their ability to do so. This finding suggests that the N7 nitrogen of guanine plays a crucial role in stabilizing triple helix formation. [, ]
Q9: How is c7dG used in DNA sequencing?
A10: c7dG is a valuable tool in DNA sequencing, particularly in resolving gel compressions caused by the formation of secondary structures in GC-rich regions. [] Replacing dGTP with this compound-5'-triphosphate (c7dGTP) during PCR or sequencing reactions helps to disrupt these structures, resulting in more uniform band intensities and improving the accuracy of base calling. [, , ]
Q10: Can c7dG be used to study the mechanism of telomerase?
A11: Yes, c7dG and its triphosphate form (7-deaza-dGTP) have been used to investigate the mechanism of telomerase, an enzyme responsible for maintaining the ends of chromosomes. Research shows that 7-deaza-dGTP acts as a substrate for telomerase but alters its processivity, resulting in the synthesis of shorter telomeric DNA products. [, ]
Q11: How does the hydration of DNA containing c7dG differ from natural DNA?
A12: Crystallographic and computational studies reveal that DNA containing c7dG, specifically a Dickerson-Drew Dodecamer (DDD) with c7dG and other modified bases, exhibits a significant decrease in hydration compared to the natural DNA sequence. This altered hydration, along with changes in major groove electrostatics, contributes to the increased conformational flexibility observed in the modified DDD. []
Q12: Can molecular modeling be used to explain the fluorescence properties of c7dG derivatives?
A13: Yes, molecular modeling techniques are instrumental in understanding the fluorescence properties of c7dG derivatives. For example, in a study involving the synthesis of a c7dG derivative with two pyrene moieties attached via a tripropargylamine linker, molecular modeling provided insights into the observed excimer fluorescence. The model indicated that only two out of the four pyrene residues in a DNA duplex were positioned close enough to interact and generate the excimer signal. []
Q13: What are the potential applications of c7dG in the development of novel therapeutics?
A14: The unique properties of c7dG make it a promising candidate for developing novel therapeutics. For example, its ability to modulate telomerase activity suggests potential applications in anti-cancer therapies. [, ] Additionally, the incorporation of c7dG into antisense oligonucleotides could enhance their stability and target specificity for gene silencing applications. [, ]
Q14: What are the limitations of using c7dG in biological systems?
A15: Despite its valuable properties, there are limitations to using c7dG in biological systems. Its incorporation can sometimes lead to decreased duplex stability depending on the sequence context. [, ] Moreover, the impact of c7dG on the fidelity of DNA replication and transcription needs further investigation to ensure its safe and effective use in biological applications.
Disclaimer and Information on In-Vitro Research Products
Please be aware that all articles and product information presented on BenchChem are intended solely for informational purposes. The products available for purchase on BenchChem are specifically designed for in-vitro studies, which are conducted outside of living organisms. In-vitro studies, derived from the Latin term "in glass," involve experiments performed in controlled laboratory settings using cells or tissues. It is important to note that these products are not categorized as medicines or drugs, and they have not received approval from the FDA for the prevention, treatment, or cure of any medical condition, ailment, or disease. We must emphasize that any form of bodily introduction of these products into humans or animals is strictly prohibited by law. It is essential to adhere to these guidelines to ensure compliance with legal and ethical standards in research and experimentation.