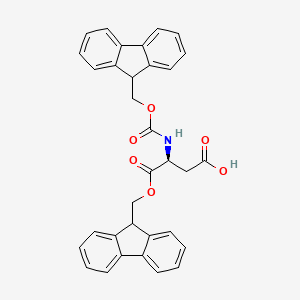
Fmoc-asp-ofm
Overview
Description
Fluorenylmethyloxycarbonyl-aspartic acid-oxalyl fluoride (Fmoc-asp-ofm) is a doubly protected aspartic acid derivative. It is widely used in peptide synthesis due to its ability to form stable and biocompatible nanostructures. The compound is particularly noted for its self-assembly properties, which make it suitable for various applications in tissue engineering and regenerative medicine .
Mechanism of Action
Target of Action
Fmoc-Asp-OFm, where Fmoc stands for fluorenylmethoxycarbonyl, is primarily used as a protecting group for amines in organic synthesis . The Fmoc group can be introduced by reacting the amine with fluorenylmethyloxycarbonyl chloride (Fmoc-Cl) .
Mode of Action
The Fmoc group acts as a base-labile protecting group . It is rapidly removed by a base, with piperidine usually preferred for Fmoc group removal as it forms a stable adduct with the dibenzofulvene byproduct, preventing it from reacting with the substrate .
Biochemical Pathways
The Fmoc group plays a significant role in Solid Phase Peptide Synthesis (SPPS), where it is used as a temporary protecting group for the amine at the N-terminus .
Result of Action
The primary result of the action of this compound is the protection of amines during peptide synthesis, allowing for the selective formation of peptide bonds . After its role is fulfilled, the Fmoc group is removed, leaving behind the desired peptide product .
Action Environment
The action of this compound is influenced by the conditions of the peptide synthesis process. For example, the Fmoc group is removed using a solution of 20% piperidine in N,N-dimethylformamide (DMF) . The efficiency of this deprotection step can be influenced by factors such as the concentration of piperidine, the temperature, and the reaction time .
Biochemical Analysis
Biochemical Properties
Fmoc-Asp-OFm plays a crucial role in biochemical reactions, particularly in the synthesis of peptides. It interacts with various enzymes and proteins involved in peptide synthesis, such as peptidyl transferases and aminoacyl-tRNA synthetases. These interactions facilitate the incorporation of aspartic acid into growing peptide chains. The Fmoc group is removed under basic conditions, while the OFm group is cleaved under acidic conditions, allowing for sequential peptide elongation .
Cellular Effects
This compound has been shown to influence various cellular processes. In particular, it has been used to create hydrogels that support cell viability, proliferation, and differentiation. These hydrogels, formed by the self-assembly of this compound, provide a scaffold for cell growth and tissue engineering applications. The compound’s ability to bind calcium ions and phosphate groups enhances its osteoinductive properties, promoting bone tissue regeneration .
Molecular Mechanism
At the molecular level, this compound exerts its effects through self-assembly into well-ordered fibrous structures. These structures serve as nucleation points for calcium and phosphate binding, facilitating the formation of hydroxyapatite, a key component of bone tissue. The Fmoc and OFm groups also protect the aspartic acid residue during peptide synthesis, preventing unwanted side reactions and ensuring the accurate incorporation of aspartic acid into peptides .
Temporal Effects in Laboratory Settings
In laboratory settings, the effects of this compound change over time. The compound is stable under standard storage conditions but can degrade under extreme pH or temperature conditions. Long-term studies have shown that this compound hydrogels maintain their structural integrity and bioactivity for extended periods, supporting sustained cell growth and differentiation .
Dosage Effects in Animal Models
The effects of this compound vary with different dosages in animal models. At low doses, the compound supports normal cellular functions and promotes tissue regeneration. At high doses, it can cause cytotoxicity and adverse effects on cellular metabolism. Threshold effects have been observed, where a specific dosage range maximizes the compound’s beneficial effects while minimizing toxicity .
Metabolic Pathways
This compound is involved in metabolic pathways related to peptide synthesis and bone tissue regeneration. It interacts with enzymes such as peptidyl transferases and aminoacyl-tRNA synthetases, facilitating the incorporation of aspartic acid into peptides. Additionally, its ability to bind calcium and phosphate ions plays a role in the formation of hydroxyapatite, a key component of bone tissue .
Transport and Distribution
Within cells and tissues, this compound is transported and distributed through interactions with specific transporters and binding proteins. These interactions influence the compound’s localization and accumulation, ensuring its availability for peptide synthesis and tissue engineering applications. The self-assembled fibrous structures formed by this compound also contribute to its distribution within the extracellular matrix .
Subcellular Localization
This compound is localized within specific subcellular compartments, where it exerts its biochemical effects. The compound’s targeting signals and post-translational modifications direct it to specific organelles, such as the endoplasmic reticulum and Golgi apparatus, where peptide synthesis occurs. Additionally, its self-assembled structures are found within the extracellular matrix, supporting tissue regeneration .
Preparation Methods
Synthetic Routes and Reaction Conditions
Fmoc-asp-ofm is typically synthesized through solid-phase peptide synthesis (SPPS). The process involves the attachment of fluorenylmethyloxycarbonyl (Fmoc) protecting groups to the aspartic acid residue. The compound is then converted to its oxalyl fluoride form. The reaction conditions often involve the use of reagents such as diisopropylethylamine (DIEA) and solvents like dimethylformamide (DMF) .
Industrial Production Methods
In industrial settings, this compound is produced in large quantities using automated peptide synthesizers. The process is optimized to ensure high purity and yield, often exceeding 99%. The compound is usually obtained in the form of a lyophilized powder .
Chemical Reactions Analysis
Types of Reactions
Fmoc-asp-ofm undergoes various chemical reactions, including:
Oxidation: The compound can be oxidized to form different derivatives.
Reduction: Reduction reactions can be used to remove the Fmoc protecting groups.
Substitution: The oxalyl fluoride group can be substituted with other functional groups.
Common Reagents and Conditions
Oxidation: Common oxidizing agents include hydrogen peroxide and potassium permanganate.
Reduction: Reducing agents like sodium borohydride are often used.
Substitution: Substitution reactions typically involve nucleophiles such as amines and alcohols.
Major Products Formed
The major products formed from these reactions include various peptide derivatives and modified aspartic acid residues. These products are often used in further peptide synthesis and biochemical applications .
Scientific Research Applications
Hydrogel Formation and Tissue Engineering
Fmoc-Asp-OFm is primarily utilized for creating hydrogels that serve as scaffolds in tissue engineering. The self-assembly of this compound leads to the formation of fibrous structures that can mimic the extracellular matrix, providing a supportive environment for cell growth and differentiation.
Mechanism of Self-Assembly
The self-assembly process involves the interaction of this compound with solvents such as dimethyl sulfoxide and water, resulting in the formation of stable hydrogels. The presence of calcium ions enhances this process, promoting nucleation sites for phosphate groups which are essential for bone tissue engineering.
Case Study: Bone Tissue Engineering
A study demonstrated that hydrogels formed from this compound exhibited osteoinductive properties. When pre-osteoblastic cells were cultured on these hydrogels, they showed significant cell viability and proliferation. The mechanical properties of these hydrogels were characterized using uniaxial compression tests, revealing a Young’s modulus comparable to that of natural cartilage (approximately 50 kPa) .
Table 1: Formation Conditions for this compound Hydrogels
Condition | Composition | Observations |
---|---|---|
Control Hydrogel | 3 mg/mL this compound in DMSO | Stable hydrogel formation |
Hydrogel with Calcium | 3 mg/mL this compound in 7 mM CaCl₂ | Enhanced mechanical stability |
Hydrogel with Calcium and Phosphate | 3 mg/mL this compound in 7 mM CaCl₂ + 0.6 M Na₂HPO₄ | Osteoinductive properties observed |
Drug Delivery Systems
The ability of this compound to form hydrogels also positions it as a promising candidate for drug delivery applications. The hydrophilic nature of these gels allows for the encapsulation of various therapeutic agents.
Encapsulation Efficiency
Research has shown that drugs can be effectively encapsulated within the hydrogel matrix formed by this compound, allowing for controlled release profiles. This feature is particularly beneficial for delivering drugs in a sustained manner over extended periods.
Peptide Synthesis
This compound is widely used in solid-phase peptide synthesis (SPPS). The fluorenyl methoxycarbonyl protecting group facilitates the sequential addition of amino acids while preventing undesired reactions.
Cyclization Strategies
Recent studies have explored various cyclization techniques involving this compound to synthesize cyclic peptides with enhanced stability and biological activity. These cyclic peptides have potential applications in drug design and development .
Table 2: Cyclization Methods Using this compound
Method | Description | Yield |
---|---|---|
Solution Cyclization | Linear precursor cyclized in solution | ~10% |
Solid Phase Cyclization | Side-chain anchoring followed by cyclization on resin | Higher yields with minimal purification required |
Market Trends and Future Directions
The market for this compound reagents is projected to grow significantly due to increasing demand in research and development sectors related to life sciences. Challenges such as high production costs remain, but advancements in synthetic methodologies are expected to mitigate these issues .
Comparison with Similar Compounds
Similar Compounds
Fmoc-aspartic acid: Similar in structure but lacks the oxalyl fluoride group.
Fmoc-glutamic acid: Another Fmoc-protected amino acid with similar self-assembly properties.
Fmoc-phenylalanine: Known for its antimicrobial properties but differs in its application spectrum.
Uniqueness
Fmoc-asp-ofm is unique due to its dual protection and ability to form stable hydrogels. This makes it particularly suitable for applications in bone tissue engineering, where mechanical stability and biocompatibility are crucial .
Biological Activity
Fmoc-Asp-OFm (Nα-fluorenylmethoxycarbonyl-L-aspartic acid) is a modified amino acid that has garnered attention in the fields of biomaterials and tissue engineering due to its unique properties. This article delves into its biological activity, particularly focusing on its applications in hydrogel formation and cellular interactions.
Overview of this compound
This compound is characterized by the presence of the Fmoc protecting group, which enhances its ability to self-assemble into hydrogels. These hydrogels are of great interest for biomedical applications, particularly in tissue engineering, where they can serve as scaffolds for cell culture and regeneration.
Hydrogel Formation and Properties
This compound can form hydrogels under specific conditions, particularly in the presence of calcium ions. The hydrogels created from this compound exhibit several advantageous properties:
- Self-Assembly : The Fmoc moiety facilitates π-π stacking interactions, leading to the formation of a fibrous network.
- Mechanical Stability : The incorporation of calcium ions enhances the mechanical properties of the hydrogels, making them suitable for load-bearing applications.
- Biocompatibility : These hydrogels support cell attachment, proliferation, and differentiation, making them ideal for tissue engineering applications.
Table 1: Properties of this compound Hydrogels
Property | Description |
---|---|
Self-Assembly Capability | Forms hydrogels through π-π stacking |
Mechanical Strength | Enhanced by calcium ion incorporation |
Biocompatibility | Supports cell viability and differentiation |
Hydrogel Structure | Fibrous network with fibril diameters ranging from 50 to 200 nm |
Biological Activity Studies
Research has demonstrated that this compound-based hydrogels promote osteogenic differentiation in pre-osteoblastic cells. A study used the MC3T3-E1 mouse calvaria osteoblastic precursor cell line to investigate the effects of these hydrogels on cell viability and differentiation.
Key Findings:
- Cell Viability : Cells cultured on this compound hydrogels showed high viability over a period of 21 days.
- Osteogenic Differentiation : Alkaline phosphatase (ALP) activity was significantly increased in cells cultured on these hydrogels compared to control groups, indicating enhanced osteogenic differentiation.
Table 2: Alkaline Phosphatase Activity Over Time
Time (Days) | ALP Activity (U/mL) |
---|---|
7 | 45 |
14 | 75 |
21 | 120 |
Mechanistic Insights
The mechanism underlying the biological activity of this compound involves several factors:
- Calcium Nucleation : The acidic nature of aspartic acid facilitates calcium binding, which is crucial for bone mineralization.
- Hydrogel Architecture : The fibrous structure provides a conducive environment for cell attachment and migration.
Case Studies
- Bone Tissue Engineering : In a study exploring bone regeneration, this compound hydrogels were implanted in critical-sized bone defects in animal models. The results indicated significant bone formation and integration with surrounding tissues.
- Drug Delivery Systems : Hydrogels formed with this compound have been utilized for controlled drug release. The encapsulation efficiency and release kinetics were optimized by varying the hydrogel composition.
Properties
IUPAC Name |
(3S)-4-(9H-fluoren-9-ylmethoxy)-3-(9H-fluoren-9-ylmethoxycarbonylamino)-4-oxobutanoic acid | |
---|---|---|
Source | PubChem | |
URL | https://pubchem.ncbi.nlm.nih.gov | |
Description | Data deposited in or computed by PubChem | |
InChI |
InChI=1S/C33H27NO6/c35-31(36)17-30(32(37)39-18-28-24-13-5-1-9-20(24)21-10-2-6-14-25(21)28)34-33(38)40-19-29-26-15-7-3-11-22(26)23-12-4-8-16-27(23)29/h1-16,28-30H,17-19H2,(H,34,38)(H,35,36)/t30-/m0/s1 | |
Source | PubChem | |
URL | https://pubchem.ncbi.nlm.nih.gov | |
Description | Data deposited in or computed by PubChem | |
InChI Key |
AAVDDDPGTRDTJT-PMERELPUSA-N | |
Source | PubChem | |
URL | https://pubchem.ncbi.nlm.nih.gov | |
Description | Data deposited in or computed by PubChem | |
Canonical SMILES |
C1=CC=C2C(=C1)C(C3=CC=CC=C32)COC(=O)C(CC(=O)O)NC(=O)OCC4C5=CC=CC=C5C6=CC=CC=C46 | |
Source | PubChem | |
URL | https://pubchem.ncbi.nlm.nih.gov | |
Description | Data deposited in or computed by PubChem | |
Isomeric SMILES |
C1=CC=C2C(=C1)C(C3=CC=CC=C32)COC(=O)[C@H](CC(=O)O)NC(=O)OCC4C5=CC=CC=C5C6=CC=CC=C46 | |
Source | PubChem | |
URL | https://pubchem.ncbi.nlm.nih.gov | |
Description | Data deposited in or computed by PubChem | |
Molecular Formula |
C33H27NO6 | |
Source | PubChem | |
URL | https://pubchem.ncbi.nlm.nih.gov | |
Description | Data deposited in or computed by PubChem | |
DSSTOX Substance ID |
DTXSID70692810 | |
Record name | (3S)-4-[(9H-Fluoren-9-yl)methoxy]-3-({[(9H-fluoren-9-yl)methoxy]carbonyl}amino)-4-oxobutanoic acid (non-preferred name) | |
Source | EPA DSSTox | |
URL | https://comptox.epa.gov/dashboard/DTXSID70692810 | |
Description | DSSTox provides a high quality public chemistry resource for supporting improved predictive toxicology. | |
Molecular Weight |
533.6 g/mol | |
Source | PubChem | |
URL | https://pubchem.ncbi.nlm.nih.gov | |
Description | Data deposited in or computed by PubChem | |
CAS No. |
187671-16-5 | |
Record name | (3S)-4-[(9H-Fluoren-9-yl)methoxy]-3-({[(9H-fluoren-9-yl)methoxy]carbonyl}amino)-4-oxobutanoic acid (non-preferred name) | |
Source | EPA DSSTox | |
URL | https://comptox.epa.gov/dashboard/DTXSID70692810 | |
Description | DSSTox provides a high quality public chemistry resource for supporting improved predictive toxicology. | |
Retrosynthesis Analysis
AI-Powered Synthesis Planning: Our tool employs the Template_relevance Pistachio, Template_relevance Bkms_metabolic, Template_relevance Pistachio_ringbreaker, Template_relevance Reaxys, Template_relevance Reaxys_biocatalysis model, leveraging a vast database of chemical reactions to predict feasible synthetic routes.
One-Step Synthesis Focus: Specifically designed for one-step synthesis, it provides concise and direct routes for your target compounds, streamlining the synthesis process.
Accurate Predictions: Utilizing the extensive PISTACHIO, BKMS_METABOLIC, PISTACHIO_RINGBREAKER, REAXYS, REAXYS_BIOCATALYSIS database, our tool offers high-accuracy predictions, reflecting the latest in chemical research and data.
Strategy Settings
Precursor scoring | Relevance Heuristic |
---|---|
Min. plausibility | 0.01 |
Model | Template_relevance |
Template Set | Pistachio/Bkms_metabolic/Pistachio_ringbreaker/Reaxys/Reaxys_biocatalysis |
Top-N result to add to graph | 6 |
Feasible Synthetic Routes
Disclaimer and Information on In-Vitro Research Products
Please be aware that all articles and product information presented on BenchChem are intended solely for informational purposes. The products available for purchase on BenchChem are specifically designed for in-vitro studies, which are conducted outside of living organisms. In-vitro studies, derived from the Latin term "in glass," involve experiments performed in controlled laboratory settings using cells or tissues. It is important to note that these products are not categorized as medicines or drugs, and they have not received approval from the FDA for the prevention, treatment, or cure of any medical condition, ailment, or disease. We must emphasize that any form of bodily introduction of these products into humans or animals is strictly prohibited by law. It is essential to adhere to these guidelines to ensure compliance with legal and ethical standards in research and experimentation.