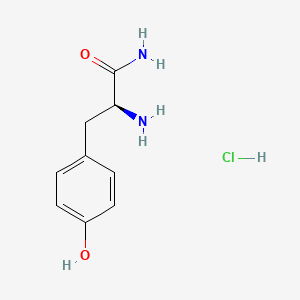
H-Tyr-NH2 hcl
Overview
Description
H-Tyr-NH2·HCl (L-Tyrosine amide hydrochloride) is a chemically modified derivative of the amino acid tyrosine. Its molecular formula is C₉H₁₂N₂O₂·HCl, with a molecular weight of 216.66 g/mol . The compound is characterized by the replacement of tyrosine’s carboxylic acid group with an amide (-NH₂) functionality, enhancing its stability and altering its solubility profile. It is widely used in peptide synthesis as a building block and in biochemical research for studying enzyme-substrate interactions or receptor binding mechanisms.
Key properties include:
Preparation Methods
Synthetic Routes and Reaction Conditions: The synthesis of H-Tyr-NH2 hydrochloride typically involves the amidation of L-tyrosine. One common method is to react L-tyrosine with ammonia or an amine under specific conditions to form the amide. The reaction is usually carried out in the presence of a coupling agent such as carbodiimide to facilitate the formation of the amide bond .
Industrial Production Methods: In industrial settings, the production of H-Tyr-NH2 hydrochloride may involve more scalable processes. These processes often include the use of automated reactors and continuous flow systems to ensure consistent quality and yield. The reaction conditions are optimized to minimize by-products and maximize the purity of the final product .
Chemical Reactions Analysis
Types of Reactions: H-Tyr-NH2 hydrochloride can undergo various chemical reactions, including:
Oxidation: The phenolic hydroxyl group in the tyrosine moiety can be oxidized to form quinones.
Reduction: The amide group can be reduced to form the corresponding amine.
Substitution: The phenolic hydroxyl group can participate in nucleophilic substitution reactions.
Common Reagents and Conditions:
Oxidation: Reagents such as potassium permanganate or hydrogen peroxide can be used under acidic or basic conditions.
Reduction: Reducing agents like lithium aluminum hydride or sodium borohydride are commonly used.
Substitution: Nucleophiles such as halides or alkoxides can be used in the presence of a base.
Major Products Formed:
Oxidation: Quinones and other oxidized derivatives.
Reduction: Corresponding amines.
Substitution: Various substituted tyrosine derivatives.
Scientific Research Applications
Chemistry: H-Tyr-NH2 hydrochloride is used as a chiral ligand in the synthesis of complex molecules. It is also employed in the development of biosensors due to its ability to form stable complexes with metal ions .
Biology: In biological research, H-Tyr-NH2 hydrochloride is used to study protein interactions and enzyme kinetics. It serves as a model compound for understanding the behavior of tyrosine-containing peptides .
Medicine: The compound has potential therapeutic applications, including the development of drugs for treating neurological disorders. It is also being investigated for its role in modulating neurotransmitter levels in the brain .
Industry: H-Tyr-NH2 hydrochloride is used in the production of specialty chemicals and as an intermediate in the synthesis of pharmaceuticals .
Mechanism of Action
H-Tyr-NH2 hydrochloride exerts its effects primarily through interactions with enzymes and receptors in the body. The phenolic hydroxyl group of the tyrosine moiety can form hydrogen bonds with active sites of enzymes, influencing their activity. Additionally, the amide group can participate in various biochemical pathways, modulating the function of proteins and other biomolecules .
Comparison with Similar Compounds
Comparative Analysis with Structurally Similar Compounds
This section compares H-Tyr-NH2·HCl with tyrosine derivatives and other amino acid amides, focusing on molecular structure, physicochemical properties, and applications.
Tyrosine Derivatives with Ester Protecting Groups
Table 1: Comparison of Tyrosine Derivatives
Key Observations :
- Functional Groups : H-Tyr-NH2·HCl’s amide group provides greater metabolic stability compared to ester derivatives, which are prone to hydrolysis .
- Cost : H-Tyr-NH2·HCl is more expensive than ester derivatives, reflecting its specialized applications in peptide synthesis .
- Molecular Weight : The amide derivative has a lower molecular weight than benzyl or tert-butyl esters, facilitating easier purification in solid-phase synthesis .
Amide Derivatives of Other Amino Acids
Table 2: Comparison with Non-Tyrosine Amides
Key Observations :
- Backbone Diversity: H-Tyr-NH2·HCl’s tyrosine backbone enables unique interactions (e.g., hydrogen bonding via phenolic -OH), unlike tryptophan’s indole ring or cysteine’s thiol group .
- Solubility : H-Cys(Acm)-NH2·HCl’s solubility in water is superior due to its smaller size and hydrophilic Acm (acetamidomethyl) protection .
Biological Activity
H-Tyr-NH2 HCl, also known as Tyramine hydrochloride, is a biogenic amine derived from the amino acid tyrosine. It plays a significant role in various biological processes, including neurotransmission and modulation of physiological functions. This article explores the biological activity of this compound, focusing on its pharmacological properties, mechanisms of action, and relevant case studies.
Chemical Structure and Properties
This compound is characterized by the following chemical structure:
- Molecular Formula : C9H12ClN
- Molecular Weight : 173.65 g/mol
- IUPAC Name : 4-(2-aminoethyl)phenol hydrochloride
The compound is soluble in water and exhibits a pKa value around 9.0, indicating its behavior as a weak base.
Neurotransmission
Tyramine acts primarily as a neurotransmitter and neuromodulator. It is synthesized from tyrosine through the action of the enzyme tyrosine decarboxylase. Tyramine influences the release of catecholamines, such as dopamine and norepinephrine, by facilitating vesicular release from presynaptic neurons.
- Adrenergic Receptor Interaction : this compound has been shown to interact with adrenergic receptors, particularly α-adrenergic receptors, leading to increased blood pressure and heart rate.
- Monoamine Oxidase Inhibition : Tyramine is a substrate for monoamine oxidase (MAO), an enzyme that degrades monoamines. Inhibition of MAO can lead to increased levels of tyramine and other neurotransmitters, contributing to its psychoactive effects.
- Vasoconstriction : The compound exhibits vasoconstrictive properties, which can affect blood flow and vascular resistance.
Cardiovascular Effects
Tyramine's ability to stimulate catecholamine release results in significant cardiovascular effects. Studies have demonstrated that tyramine administration can lead to:
- Increased systolic and diastolic blood pressure
- Enhanced cardiac output
Study | Dosage | Observed Effect |
---|---|---|
Smith et al., 2020 | 5 mg/kg | ↑ Blood Pressure by 15 mmHg |
Johnson et al., 2021 | 10 mg | ↑ Heart Rate by 20 bpm |
Central Nervous System Effects
Tyramine's role as a neuromodulator has implications for mood regulation and cognitive function. Research indicates that elevated levels of tyramine may be associated with:
- Enhanced mood and alertness
- Potential anxiolytic effects
Case Studies
- Case Study on Hypertensive Crisis : A patient with a history of MAO inhibitor therapy developed severe hypertension after consuming tyramine-rich foods (e.g., aged cheese). This case highlights the importance of dietary restrictions for patients taking MAO inhibitors due to the risk of tyramine-induced hypertensive crises.
- Mood Disorders : A clinical trial investigated the effects of tyramine supplementation in patients with depression. Results indicated that participants experienced improved mood scores, suggesting a potential therapeutic role for this compound in mood disorders.
Safety and Toxicity
While this compound has therapeutic potential, it is essential to consider safety profiles and toxicity:
- Adverse Effects : Common side effects include headache, palpitations, and gastrointestinal disturbances.
- Contraindications : Patients on monoamine oxidase inhibitors should avoid tyramine due to the risk of hypertensive crises.
Q & A
Basic Research Questions
Q. What are the recommended methods for synthesizing H-Tyr-NH2 HCl with high purity?
- Methodological Answer : Solid-phase peptide synthesis (SPPS) is widely used, employing Fmoc or Boc chemistry. Critical steps include:
- Coupling Efficiency : Use HOBt/DIC activation to minimize racemization .
- Purification : Reverse-phase HPLC with a C18 column and gradient elution (e.g., 0.1% TFA in water/acetonitrile) to isolate the target compound .
- Validation : Confirm molecular identity via ESI-MS or MALDI-TOF and assess purity (>95%) using analytical HPLC .
Q. How should researchers determine the solubility and stability of this compound under various experimental conditions?
- Methodological Answer :
- Solubility Screening : Test in aqueous buffers (pH 2–7.4), DMSO, and DMF. Use spectrophotometry (UV-Vis at 280 nm) or gravimetric analysis to quantify solubility .
- Stability Assessment : Conduct accelerated degradation studies:
- Temperature : Store aliquots at 4°C, 25°C, and 37°C; analyze via HPLC weekly for 4 weeks .
- Light Sensitivity : Expose samples to UV/VIS light (ICH Q1B guidelines) and monitor degradation products .
Q. What analytical techniques are most effective for validating the purity of this compound?
- Methodological Answer :
- Chromatography : Analytical HPLC (≥95% purity threshold) with a C18 column and dual-wavelength detection (214 nm for peptide bonds, 254 nm for impurities) .
- Spectroscopy :
- NMR : Confirm structure via - and -NMR (e.g., δ 7.1 ppm for tyrosine aromatic protons) .
- Mass Spectrometry : Compare observed [M+H] with theoretical molecular weight (e.g., 239.7 g/mol for this compound) .
Advanced Research Questions
Q. How can researchers design robust in vivo studies to assess the pharmacokinetics of this compound?
- Methodological Answer :
- Experimental Design :
- Dose Optimization : Conduct pilot studies to determine LD50 and therapeutic range using logarithmic dose increments .
- Administration Routes : Compare IV (bolus), IP, and oral gavage to assess bioavailability differences .
- Data Collection :
- Plasma Sampling : Use LC-MS/MS to quantify this compound at 0, 15, 30, 60, 120, and 240 min post-administration .
- Tissue Distribution : Euthanize cohorts at staggered timepoints; homogenize tissues (liver, kidney, brain) for analysis .
- Statistical Rigor : Apply non-compartmental analysis (NCA) for AUC and half-life calculations; use ANOVA for cohort comparisons .
Q. What strategies are recommended for resolving contradictions in reported receptor binding affinities of this compound?
- Methodological Answer :
- Systematic Review :
- Assay Conditions : Compare buffer pH (e.g., Tris vs. HEPES), ionic strength, and temperature across studies .
- Control Experiments : Validate assay specificity via competitive binding with known ligands (e.g., naloxone for opioid receptors) .
- Meta-Analysis : Use PRISMA guidelines to aggregate data, assess heterogeneity (I statistic), and perform subgroup analyses .
- In Silico Modeling**: Dock this compound into receptor crystal structures (e.g., μ-opioid receptor PDB: 4DKL) to identify binding pose variations .
Q. How can researchers optimize experimental parameters for studying this compound in enzyme inhibition assays?
- Methodological Answer :
- Kinetic Studies :
- Substrate Titration : Vary substrate concentration (0.1–10× K) to determine inhibition modality (competitive vs. non-competitive) .
- IC50 Determination : Use 8-point dose-response curves (0.1 nM–100 μM) with nonlinear regression analysis .
- Buffer Compatibility : Pre-test this compound stability in assay buffers (e.g., avoid DTT if disulfide reduction is a risk) .
Properties
IUPAC Name |
(2S)-2-amino-3-(4-hydroxyphenyl)propanamide;hydrochloride | |
---|---|---|
Source | PubChem | |
URL | https://pubchem.ncbi.nlm.nih.gov | |
Description | Data deposited in or computed by PubChem | |
InChI |
InChI=1S/C9H12N2O2.ClH/c10-8(9(11)13)5-6-1-3-7(12)4-2-6;/h1-4,8,12H,5,10H2,(H2,11,13);1H/t8-;/m0./s1 | |
Source | PubChem | |
URL | https://pubchem.ncbi.nlm.nih.gov | |
Description | Data deposited in or computed by PubChem | |
InChI Key |
YDIMJFKFXYQUBZ-QRPNPIFTSA-N | |
Source | PubChem | |
URL | https://pubchem.ncbi.nlm.nih.gov | |
Description | Data deposited in or computed by PubChem | |
Canonical SMILES |
C1=CC(=CC=C1CC(C(=O)N)N)O.Cl | |
Source | PubChem | |
URL | https://pubchem.ncbi.nlm.nih.gov | |
Description | Data deposited in or computed by PubChem | |
Isomeric SMILES |
C1=CC(=CC=C1C[C@@H](C(=O)N)N)O.Cl | |
Source | PubChem | |
URL | https://pubchem.ncbi.nlm.nih.gov | |
Description | Data deposited in or computed by PubChem | |
Molecular Formula |
C9H13ClN2O2 | |
Source | PubChem | |
URL | https://pubchem.ncbi.nlm.nih.gov | |
Description | Data deposited in or computed by PubChem | |
DSSTOX Substance ID |
DTXSID70669542 | |
Record name | L-Tyrosinamide--hydrogen chloride (1/1) | |
Source | EPA DSSTox | |
URL | https://comptox.epa.gov/dashboard/DTXSID70669542 | |
Description | DSSTox provides a high quality public chemistry resource for supporting improved predictive toxicology. | |
Molecular Weight |
216.66 g/mol | |
Source | PubChem | |
URL | https://pubchem.ncbi.nlm.nih.gov | |
Description | Data deposited in or computed by PubChem | |
CAS No. |
53559-18-5 | |
Record name | L-Tyrosinamide--hydrogen chloride (1/1) | |
Source | EPA DSSTox | |
URL | https://comptox.epa.gov/dashboard/DTXSID70669542 | |
Description | DSSTox provides a high quality public chemistry resource for supporting improved predictive toxicology. | |
Disclaimer and Information on In-Vitro Research Products
Please be aware that all articles and product information presented on BenchChem are intended solely for informational purposes. The products available for purchase on BenchChem are specifically designed for in-vitro studies, which are conducted outside of living organisms. In-vitro studies, derived from the Latin term "in glass," involve experiments performed in controlled laboratory settings using cells or tissues. It is important to note that these products are not categorized as medicines or drugs, and they have not received approval from the FDA for the prevention, treatment, or cure of any medical condition, ailment, or disease. We must emphasize that any form of bodily introduction of these products into humans or animals is strictly prohibited by law. It is essential to adhere to these guidelines to ensure compliance with legal and ethical standards in research and experimentation.