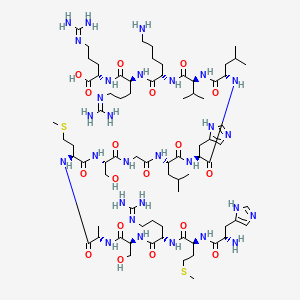
SAMS Peptide
Overview
Description
SAMS Peptide is a modified version of the sequence around the AMPK target site in rat acetyl-CoA carboxylase . It is primarily used in Kinase Assays . It is designed to mimic the sequence of the AMP-activated protein kinase (AMPK) binding site of A77S-substituted acetyl CoA carboxylase .
Synthesis Analysis
Peptide-based self-assembled monolayers (SAMs) represent highly ordered molecular materials with versatile biochemical features and multidisciplinary applications . Peptides, presenting increasing structural complexity, stimuli-responsiveness, and biological relevance, represent versatile functional components in SAMs-based platforms .
Molecular Structure Analysis
The molecular structure of this compound is complex and versatile. It is part of self-assembled monolayers (SAMs), which are highly ordered molecular materials . The peptide displays increasing structural complexity and stimuli-responsiveness .
Chemical Reactions Analysis
The chemical reactions involving this compound are complex and involve various biochemical features. The peptide is part of self-assembled monolayers (SAMs), which have made much progress since the early beginnings of Au substrates and alkanethiols .
Physical and Chemical Properties Analysis
The physical and chemical properties of this compound are determined by its structure and the conditions under which it is used. The peptide forms part of self-assembled monolayers (SAMs), which are highly ordered molecular materials .
Scientific Research Applications
Antimicrobial Properties and Design
SAMS peptide has been recognized for its potential in antimicrobial applications. Synthetic antimicrobial peptides (SAMPs) are emerging as new agents to combat infections caused by multi-drug resistant pathogens. The design of SAMPs considers their natural counterparts, aiming to enhance drug activity and effectiveness against various microbes. Advances in chemical synthesis have reduced production costs, making SAMPs a promising avenue in safeguarding human and animal health (Lima et al., 2021).
Biofouling Resistance
Research has explored the use of zwitterionic peptide monolayers, including SAMS, for antifouling purposes. These monolayers are effective against various biofouling agents, although their effectiveness can vary with specific applications, such as in barnacle larvae settlement (Ederth et al., 2018).
Role in Ribosomally Synthesized and Post-translationally Modified Peptides (RiPPs)
SAMS peptides play a crucial role in the biosynthesis of RiPPs. RiPPs have applications in antibiotic and anticancer therapies due to their unique biological properties. Radical SAM enzymes involved in RiPP biosynthesis catalyze a wide range of post-translational modifications, expanding the possibilities for natural product development (Benjdia et al., 2017).
Application in Bioengineering and Nanotechnology
SAMS peptides are utilized in bioengineering, specifically in the formation of self-assembled monolayers (SAMs) on gold surfaces. These monolayers have applications in biosensing, nanotechnology, and the development of bioactive surfaces for various medical and technological purposes (Kaplan et al., 2013).
Enzyme Assays and Biosensors
The use of SAMS peptides extends to enzyme assays and biosensors. For instance, microfluidic networks combined with peptide arrays allow for multi-enzyme assays on SAMs, demonstrating the flexibility of SAMS peptides in biological sample analysis (Su et al., 2005).
Insights into Peptide Modification and Biosynthesis
Studies on SAMS peptides provide insights into the catalysis and modification of bacterial peptides, expanding our understanding of biochemical processes and the potential for developing novel therapeutic agents (Benjdia et al., 2017).
Mechanism of Action
Target of Action
The primary target of the SAMS Peptide is the AMP-activated protein kinase (AMPK). The peptide is a substrate for this kinase and is rapidly phosphorylated by it . AMPK plays a crucial role in cellular energy homeostasis and is activated in response to stresses that deplete cellular ATP supplies such as low glucose, hypoxia, ischemia, and heat shock .
Mode of Action
The this compound interacts with AMPK by serving as a substrate for phosphorylation . This interaction results in the activation of AMPK, which then goes on to phosphorylate a range of downstream targets, thereby regulating metabolic pathways to maintain energy homeostasis .
Biochemical Pathways
The activation of AMPK by the this compound affects several biochemical pathways. AMPK acts as a metabolic master switch regulating several intracellular systems including the cellular uptake of glucose, the β-oxidation of fatty acids and the biogenesis of glucose transporter 4 (GLUT4) and mitochondria .
Pharmacokinetics
The pharmacokinetics of therapeutic peptides and proteins, including the this compound, involves processes affecting their absorption, distribution, metabolism, and excretion . These processes determine the concentration of the peptide in body fluids, preferably plasma or blood, following administration of a certain dosage regimen . Understanding these properties is crucial for designing effective dosage regimens and for the rational clinical application of the peptide .
Result of Action
The molecular and cellular effects of the action of the this compound are primarily related to its role in activating AMPK and thereby regulating energy homeostasis . This can have several downstream effects, including selective cell adhesion, migration and differentiation, biomolecular binding, advanced biosensing, molecular electronics, antimicrobial, osteointegrative and antifouling surfaces .
Action Environment
The action, efficacy, and stability of the this compound can be influenced by various environmental factors. For instance, the peptide’s function can be affected by the presence of other molecules in its environment, the pH, temperature, and ionic strength of the solution it is in . Furthermore, the peptide can form self-assembled monolayers (SAMs) that exhibit highly ordered molecular materials with versatile biochemical features and multidisciplinary applications . These SAMs can interact with various entities, including extracellular matrix components, mammalian cells, metal ions, and biocatalytic targets .
Future Directions
Peptide-based self-assembled monolayers (SAMs) have versatile biochemical features and multidisciplinary applications . They are used in various fields such as selective cell adhesion, migration and differentiation, biomolecular binding, advanced biosensing, molecular electronics, antimicrobial, osteointegrative and antifouling surfaces, among others . The future directions of SAMS Peptide research will likely continue to explore these applications and discover new ones.
Biochemical Analysis
Biochemical Properties
SAMS Peptide plays a crucial role in biochemical reactions, particularly in the formation of self-assembled monolayers. It interacts with various enzymes, proteins, and other biomolecules, facilitating selective cell adhesion, migration, and differentiation. The peptide’s interactions with biomolecules are highly specific, often involving binding to cell surface receptors or other proteins, which can trigger downstream signaling pathways .
Cellular Effects
This compound significantly influences cellular processes, including cell signaling pathways, gene expression, and cellular metabolism. It has been shown to promote cell adhesion and migration, which are essential for tissue engineering and regenerative medicine applications. Additionally, this compound can modulate gene expression by interacting with transcription factors or other regulatory proteins, thereby affecting cellular metabolism and function .
Molecular Mechanism
At the molecular level, this compound exerts its effects through specific binding interactions with biomolecules. These interactions can lead to enzyme inhibition or activation, depending on the context. For example, this compound can bind to cell surface receptors, triggering a cascade of intracellular signaling events that result in changes in gene expression and cellular behavior. Additionally, the peptide’s ability to form self-assembled monolayers enhances its stability and functionality in various biochemical applications .
Temporal Effects in Laboratory Settings
In laboratory settings, the effects of this compound can change over time. The peptide’s stability and degradation are critical factors that influence its long-term effects on cellular function. Studies have shown that this compound maintains its structural integrity and functionality over extended periods, making it suitable for long-term in vitro and in vivo studies. The peptide’s effects may diminish over time due to gradual degradation or changes in its biochemical environment .
Dosage Effects in Animal Models
The effects of this compound vary with different dosages in animal models. At lower doses, the peptide can promote beneficial cellular responses, such as enhanced cell adhesion and migration. At higher doses, this compound may exhibit toxic or adverse effects, including cytotoxicity or disruption of normal cellular functions. It is essential to determine the optimal dosage to achieve the desired therapeutic effects while minimizing potential side effects .
Metabolic Pathways
This compound is involved in various metabolic pathways, interacting with enzymes and cofactors that regulate cellular metabolism. The peptide can influence metabolic flux and metabolite levels by modulating enzyme activity or gene expression. For example, this compound may enhance the activity of enzymes involved in energy production or biosynthetic pathways, thereby supporting cellular growth and function .
Transport and Distribution
Within cells and tissues, this compound is transported and distributed through specific transporters or binding proteins. These interactions facilitate the peptide’s localization and accumulation in target cells or tissues, enhancing its therapeutic potential. The peptide’s distribution can also be influenced by its physicochemical properties, such as size, charge, and hydrophobicity .
Subcellular Localization
This compound’s subcellular localization is critical for its activity and function. The peptide can be directed to specific compartments or organelles through targeting signals or post-translational modifications. For example, this compound may localize to the cell membrane, cytoplasm, or nucleus, depending on its functional role. This localization is essential for the peptide’s ability to interact with specific biomolecules and exert its biochemical effects .
Properties
IUPAC Name |
(2S)-2-[[(2S)-2-[[(2S)-6-amino-2-[[(2S)-2-[[(2S)-2-[[(2S)-2-[[(2S)-2-[[2-[[(2S)-2-[[(2S)-2-[[(2S)-2-[[(2S)-2-[[(2S)-2-[[(2S)-2-[[(2S)-2-amino-3-(1H-imidazol-5-yl)propanoyl]amino]-4-methylsulfanylbutanoyl]amino]-5-(diaminomethylideneamino)pentanoyl]amino]-3-hydroxypropanoyl]amino]propanoyl]amino]-4-methylsulfanylbutanoyl]amino]-3-hydroxypropanoyl]amino]acetyl]amino]-4-methylpentanoyl]amino]-3-(1H-imidazol-5-yl)propanoyl]amino]-4-methylpentanoyl]amino]-3-methylbutanoyl]amino]hexanoyl]amino]-5-(diaminomethylideneamino)pentanoyl]amino]-5-(diaminomethylideneamino)pentanoic acid | |
---|---|---|
Source | PubChem | |
URL | https://pubchem.ncbi.nlm.nih.gov | |
Description | Data deposited in or computed by PubChem | |
InChI |
InChI=1S/C74H131N29O18S2/c1-38(2)27-51(66(115)100-53(30-43-32-84-37-90-43)67(116)99-52(28-39(3)4)68(117)103-57(40(5)6)70(119)97-45(15-10-11-21-75)61(110)95-46(16-12-22-85-72(77)78)62(111)98-50(71(120)121)18-14-24-87-74(81)82)92-56(106)33-88-60(109)54(34-104)101-65(114)48(19-25-122-8)93-58(107)41(7)91-69(118)55(35-105)102-63(112)47(17-13-23-86-73(79)80)96-64(113)49(20-26-123-9)94-59(108)44(76)29-42-31-83-36-89-42/h31-32,36-41,44-55,57,104-105H,10-30,33-35,75-76H2,1-9H3,(H,83,89)(H,84,90)(H,88,109)(H,91,118)(H,92,106)(H,93,107)(H,94,108)(H,95,110)(H,96,113)(H,97,119)(H,98,111)(H,99,116)(H,100,115)(H,101,114)(H,102,112)(H,103,117)(H,120,121)(H4,77,78,85)(H4,79,80,86)(H4,81,82,87)/t41-,44-,45-,46-,47-,48-,49-,50-,51-,52-,53-,54-,55-,57-/m0/s1 | |
Source | PubChem | |
URL | https://pubchem.ncbi.nlm.nih.gov | |
Description | Data deposited in or computed by PubChem | |
InChI Key |
YJAHUEAICBOBTC-CSVPVIMBSA-N | |
Source | PubChem | |
URL | https://pubchem.ncbi.nlm.nih.gov | |
Description | Data deposited in or computed by PubChem | |
Canonical SMILES |
CC(C)CC(C(=O)NC(CC1=CN=CN1)C(=O)NC(CC(C)C)C(=O)NC(C(C)C)C(=O)NC(CCCCN)C(=O)NC(CCCN=C(N)N)C(=O)NC(CCCN=C(N)N)C(=O)O)NC(=O)CNC(=O)C(CO)NC(=O)C(CCSC)NC(=O)C(C)NC(=O)C(CO)NC(=O)C(CCCN=C(N)N)NC(=O)C(CCSC)NC(=O)C(CC2=CN=CN2)N | |
Source | PubChem | |
URL | https://pubchem.ncbi.nlm.nih.gov | |
Description | Data deposited in or computed by PubChem | |
Isomeric SMILES |
C[C@@H](C(=O)N[C@@H](CCSC)C(=O)N[C@@H](CO)C(=O)NCC(=O)N[C@@H](CC(C)C)C(=O)N[C@@H](CC1=CN=CN1)C(=O)N[C@@H](CC(C)C)C(=O)N[C@@H](C(C)C)C(=O)N[C@@H](CCCCN)C(=O)N[C@@H](CCCN=C(N)N)C(=O)N[C@@H](CCCN=C(N)N)C(=O)O)NC(=O)[C@H](CO)NC(=O)[C@H](CCCN=C(N)N)NC(=O)[C@H](CCSC)NC(=O)[C@H](CC2=CN=CN2)N | |
Source | PubChem | |
URL | https://pubchem.ncbi.nlm.nih.gov | |
Description | Data deposited in or computed by PubChem | |
Molecular Formula |
C74H131N29O18S2 | |
Source | PubChem | |
URL | https://pubchem.ncbi.nlm.nih.gov | |
Description | Data deposited in or computed by PubChem | |
Molecular Weight |
1779.2 g/mol | |
Source | PubChem | |
URL | https://pubchem.ncbi.nlm.nih.gov | |
Description | Data deposited in or computed by PubChem | |
Disclaimer and Information on In-Vitro Research Products
Please be aware that all articles and product information presented on BenchChem are intended solely for informational purposes. The products available for purchase on BenchChem are specifically designed for in-vitro studies, which are conducted outside of living organisms. In-vitro studies, derived from the Latin term "in glass," involve experiments performed in controlled laboratory settings using cells or tissues. It is important to note that these products are not categorized as medicines or drugs, and they have not received approval from the FDA for the prevention, treatment, or cure of any medical condition, ailment, or disease. We must emphasize that any form of bodily introduction of these products into humans or animals is strictly prohibited by law. It is essential to adhere to these guidelines to ensure compliance with legal and ethical standards in research and experimentation.