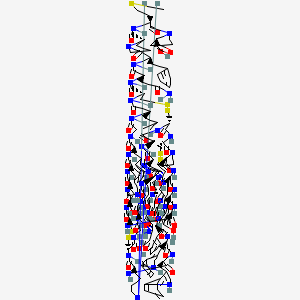
Guangxitoxin 1E
- Click on QUICK INQUIRY to receive a quote from our team of experts.
- With the quality product at a COMPETITIVE price, you can focus more on your research.
Overview
Description
Guangxitoxin 1E is a peptide toxin derived from the venom of the tarantula Plesiophrictus guangxiensis. It is known for its potent ability to inhibit voltage-gated potassium channels, specifically KV2.1 and KV2.2 channels
Mechanism of Action
Target of Action
Guangxitoxin 1E primarily targets the voltage-gated Kv2.1 potassium channels . These channels are prominently expressed in pancreatic β-cells . The Kv2.1 channels play a crucial role in regulating the electrical activity of the cells, and thus, their function .
Mode of Action
This compound acts as a gating modifier, shifting the voltage-dependence of Kv2.1 potassium currents towards depolarized potentials . It inhibits Kv2.1 channels by binding to the voltage sensors of the channel and acting as a partial inverse agonist . When bound to this compound, Kv2.1 activates more slowly, deactivates more rapidly, and requires more positive voltage to reach the same potassium conductance as the unbound channel .
Biochemical Pathways
By inhibiting Kv2.1 potassium channels, this compound boosts action potentials of pancreatic β-cells . This leads to increased glucose-stimulated intracellular calcium oscillations . The rise in intracellular calcium levels triggers the exocytosis of insulin granules, intensifying glucose-stimulated insulin secretion .
Pharmacokinetics
It is known that this compound enhances glucose-dependent insulin secretion from mouse islets . The half-maximal effective concentration (EC50) is about 400 nM in intact islets, roughly 100-fold higher than the half-maximal inhibitory concentration (IC50) for human Kv2.1 inhibition . This suggests that the bioavailability of this compound may be influenced by factors such as its concentration and the presence of glucose.
Result of Action
The primary result of this compound’s action is the enhancement of insulin secretion from pancreatic β-cells . By inhibiting Kv2.1 channels and boosting action potentials, this compound increases glucose-stimulated intracellular calcium oscillations, which in turn intensifies glucose-stimulated insulin secretion .
Action Environment
The action of this compound is influenced by the cellular and physiological environment. For instance, the presence of glucose is necessary for this compound to enhance insulin secretion . Additionally, the effect of this compound on Kv2.1 channels is voltage-dependent Therefore, the membrane potential of the cell can influence the efficacy of this compound
Biochemical Analysis
Biochemical Properties
Guangxitoxin 1E plays a significant role in biochemical reactions by inhibiting voltage-gated potassium channels, specifically KV2.1 and KV2.2 channels. This inhibition is achieved through the binding of this compound to the voltage-sensing domain of these channels, thereby altering their gating properties. The interaction between this compound and the KV2.1 channel involves the modification of the voltage-dependent gating, which reduces the affinity of the channel for the toxin . This interaction is crucial for understanding the role of this compound in modulating potassium ion flux across cell membranes.
Cellular Effects
This compound exerts various effects on different types of cells and cellular processes. In pancreatic β-cells, this compound enhances glucose-stimulated insulin secretion by inhibiting the delayed rectifier potassium current . This inhibition prolongs action potentials and increases intracellular calcium oscillations, which are essential for insulin secretion. Additionally, this compound has been shown to increase plasma somatostatin levels in mice without significantly affecting plasma insulin, glucagon, or blood glucose levels . These cellular effects highlight the potential therapeutic applications of this compound in regulating insulin secretion and glucose homeostasis.
Molecular Mechanism
The molecular mechanism of this compound involves its binding to the voltage sensors of the KV2.1 channel, acting as a partial inverse agonist . This binding stabilizes the voltage sensors in their resting conformation, thereby inhibiting the channel’s activation. This compound reduces the probability of voltage sensors activating, which limits the opportunities for the channel pore to open . This mechanism results in decreased potassium ion conductance and altered cellular excitability. The detailed understanding of this molecular mechanism provides insights into the potential therapeutic applications of this compound in modulating ion channel activity.
Temporal Effects in Laboratory Settings
In laboratory settings, the effects of this compound have been observed to change over time. The stability and degradation of this compound are critical factors influencing its long-term effects on cellular function. Studies have shown that this compound can inhibit KV2.1 channel currents in mouse acute cortical brain slices, with the inhibition being sustained over time . Additionally, the temporal effects of this compound on insulin secretion have been studied, demonstrating its ability to enhance glucose-dependent insulin secretion from mouse islets . These findings highlight the importance of considering the temporal dynamics of this compound in experimental settings.
Dosage Effects in Animal Models
The effects of this compound vary with different dosages in animal models. At low doses, this compound enhances glucose-stimulated insulin secretion without causing significant adverse effects . At higher doses, this compound may exhibit toxic effects, including prolonged action potentials and increased intracellular calcium oscillations . These dosage-dependent effects underscore the importance of optimizing the dosage of this compound for therapeutic applications while minimizing potential toxicity.
Metabolic Pathways
This compound is involved in metabolic pathways that regulate potassium ion flux and insulin secretion. The inhibition of KV2.1 channels by this compound shifts the voltage dependence of channel activation to more depolarized potentials, a characteristic of gating-modifier peptides . This shift in voltage dependence enhances glucose-stimulated intracellular calcium oscillations and insulin secretion from pancreatic β-cells . Understanding the metabolic pathways involving this compound provides valuable insights into its potential therapeutic applications in diabetes and other metabolic disorders.
Transport and Distribution
The transport and distribution of this compound within cells and tissues are critical for its biological activity. This compound is transported across cell membranes and distributed within various tissues, including the pancreas and brain . The interaction of this compound with specific transporters and binding proteins influences its localization and accumulation within cells . These transport and distribution mechanisms are essential for understanding the pharmacokinetics and pharmacodynamics of this compound.
Subcellular Localization
This compound exhibits specific subcellular localization patterns that influence its activity and function. In pancreatic β-cells, this compound is localized to the plasma membrane, where it interacts with KV2.1 channels to modulate potassium ion flux and insulin secretion . The subcellular localization of this compound is influenced by targeting signals and post-translational modifications that direct it to specific compartments or organelles . Understanding the subcellular localization of this compound provides insights into its mechanism of action and potential therapeutic applications.
Preparation Methods
Synthetic Routes and Reaction Conditions: Guangxitoxin 1E is synthesized using solid-phase peptide synthesis, a method that allows for the sequential addition of amino acids to a growing peptide chain. The synthesis involves the use of protected amino acids, which are deprotected and coupled in a specific sequence to form the desired peptide . The reaction conditions typically include the use of a resin-bound peptide, coupling reagents such as N,N’-diisopropylcarbodiimide, and deprotection agents like trifluoroacetic acid.
Industrial Production Methods: Industrial production of this compound follows similar principles as laboratory synthesis but on a larger scale. The process involves automated peptide synthesizers, which can handle multiple peptide sequences simultaneously. The synthesized peptide is then purified using high-performance liquid chromatography to achieve high purity levels .
Chemical Reactions Analysis
Types of Reactions: Guangxitoxin 1E primarily undergoes peptide bond formation and disulfide bond formation during its synthesis. The peptide bonds are formed through nucleophilic substitution reactions, while the disulfide bonds are formed through oxidation reactions .
Common Reagents and Conditions:
Peptide Bond Formation: N,N’-diisopropylcarbodiimide, hydroxybenzotriazole, and protected amino acids.
Disulfide Bond Formation: Oxidizing agents such as iodine or air oxidation under basic conditions.
Major Products: The major product of these reactions is the fully synthesized and folded peptide toxin, this compound, with its characteristic disulfide bonds that stabilize its structure .
Scientific Research Applications
Guangxitoxin 1E has a wide range of applications in scientific research:
Chemistry: It is used as a tool to study the structure and function of voltage-gated potassium channels.
Comparison with Similar Compounds
Hanatoxin: Another tarantula toxin that inhibits KV2.1 channels but also affects other potassium channels.
Stromatoxin: A peptide toxin that targets KV2.1 and KV2.2 channels with similar potency.
Jingzhaotoxin-III: A toxin with sequence similarities to Guangxitoxin 1E but different selectivity for potassium channels.
Uniqueness: this compound is unique in its high selectivity and potency for KV2.1 and KV2.2 channels. Its ability to enhance insulin secretion by modulating potassium channel activity sets it apart from other similar compounds .
Properties
IUPAC Name |
1-[2-[[1-[2-[[4-amino-4-oxo-2-[[13,42,57,89-tetrakis(4-aminobutyl)-75-[[2-[[2-[(2-amino-4-carboxybutanoyl)amino]acetyl]amino]-4-carboxybutanoyl]amino]-66-benzyl-4,48-bis(hydroxymethyl)-92-[(4-hydroxyphenyl)methyl]-16,60,63-tris(1H-indol-3-ylmethyl)-33-methyl-22-(2-methylpropyl)-2,3a,5,11,14,17,20,23,32,35,41,44,47,50,53,56,59,62,65,68,71,74,81,87,90,93,96-heptacosaoxo-95-propan-2-yl-a,27,28,77,78,99-hexathia-2a,3,6,12,15,18,21,24,31,34,40,43,46,49,52,55,58,61,64,67,70,73,82,88,91,94,97-heptacosazahexacyclo[52.43.4.230,80.06,10.036,40.082,86]trihectane-25-carbonyl]amino]butanoyl]amino]-3-phenylpropanoyl]pyrrolidine-2-carbonyl]amino]-4-methylsulfanylbutanoyl]pyrrolidine-2-carboxylic acid |
Source
|
---|---|---|
Source | PubChem | |
URL | https://pubchem.ncbi.nlm.nih.gov | |
Description | Data deposited in or computed by PubChem | |
InChI |
InChI=1S/C178H248N44O45S7/c1-95(2)70-118-159(247)214-131(165(253)208-124(77-139(184)226)163(251)209-125(73-99-34-11-8-12-35-99)175(263)219-65-28-49-137(219)171(259)203-117(59-69-268-6)174(262)222-68-31-50-138(222)178(266)267)92-272-271-91-130-167(255)216-133-94-274-270-90-128(213-158(246)115(56-58-147(235)236)195-142(229)83-189-150(238)108(183)55-57-146(233)234)153(241)190-81-140(227)188-82-141(228)198-119(71-98-32-9-7-10-33-98)160(248)206-123(76-103-80-187-111-41-18-15-38-107(103)111)162(250)207-122(75-102-79-186-110-40-17-14-37-106(102)110)161(249)200-112(42-19-23-60-179)157(245)212-129(154(242)193-86-145(232)199-126(87-223)152(240)192-84-143(230)196-116(45-22-26-63-182)173(261)218-64-27-46-134(218)168(256)194-97(5)149(237)211-130)89-269-273-93-132(215-172(260)148(96(3)4)217-164(252)120(72-100-51-53-104(225)54-52-100)204-155(243)113(43-20-24-61-180)201-170(258)136-48-30-67-221(136)177(133)265)166(254)210-127(88-224)176(264)220-66-29-47-135(220)169(257)202-114(44-21-25-62-181)156(244)205-121(151(239)191-85-144(231)197-118)74-101-78-185-109-39-16-13-36-105(101)109/h7-18,32-41,51-54,78-80,95-97,108,112-138,148,185-187,223-225H,19-31,42-50,55-77,81-94,179-183H2,1-6H3,(H2,184,226)(H,188,227)(H,189,238)(H,190,241)(H,191,239)(H,192,240)(H,193,242)(H,194,256)(H,195,229)(H,196,230)(H,197,231)(H,198,228)(H,199,232)(H,200,249)(H,201,258)(H,202,257)(H,203,259)(H,204,243)(H,205,244)(H,206,248)(H,207,250)(H,208,253)(H,209,251)(H,210,254)(H,211,237)(H,212,245)(H,213,246)(H,214,247)(H,215,260)(H,216,255)(H,217,252)(H,233,234)(H,235,236)(H,266,267) |
Source
|
Source | PubChem | |
URL | https://pubchem.ncbi.nlm.nih.gov | |
Description | Data deposited in or computed by PubChem | |
InChI Key |
SZVUKNYCMKWHNH-UHFFFAOYSA-N |
Source
|
Source | PubChem | |
URL | https://pubchem.ncbi.nlm.nih.gov | |
Description | Data deposited in or computed by PubChem | |
Canonical SMILES |
CC1C(=O)NC2CSSCC(NC(=O)C(NC(=O)CNC(=O)C(NC(=O)C(NC(=O)C3CCCN3C(=O)C(NC(=O)C4CSSCC(C(=O)NCC(=O)NC(C(=O)NCC(=O)NC(C(=O)N5CCCC5C(=O)N1)CCCCN)CO)NC(=O)C(NC(=O)C(NC(=O)C(NC(=O)C(NC(=O)CNC(=O)CNC(=O)C(CSSCC(C(=O)N6CCCC6C(=O)NC(C(=O)NC(C(=O)NC(C(=O)N4)C(C)C)CC7=CC=C(C=C7)O)CCCCN)NC2=O)NC(=O)C(CCC(=O)O)NC(=O)CNC(=O)C(CCC(=O)O)N)CC8=CC=CC=C8)CC9=CNC1=CC=CC=C19)CC1=CNC2=CC=CC=C21)CCCCN)CO)CCCCN)CC1=CNC2=CC=CC=C21)CC(C)C)C(=O)NC(CC(=O)N)C(=O)NC(CC1=CC=CC=C1)C(=O)N1CCCC1C(=O)NC(CCSC)C(=O)N1CCCC1C(=O)O |
Source
|
Source | PubChem | |
URL | https://pubchem.ncbi.nlm.nih.gov | |
Description | Data deposited in or computed by PubChem | |
Molecular Formula |
C178H248N44O45S7 |
Source
|
Source | PubChem | |
URL | https://pubchem.ncbi.nlm.nih.gov | |
Description | Data deposited in or computed by PubChem | |
Molecular Weight |
3949 g/mol |
Source
|
Source | PubChem | |
URL | https://pubchem.ncbi.nlm.nih.gov | |
Description | Data deposited in or computed by PubChem | |
Disclaimer and Information on In-Vitro Research Products
Please be aware that all articles and product information presented on BenchChem are intended solely for informational purposes. The products available for purchase on BenchChem are specifically designed for in-vitro studies, which are conducted outside of living organisms. In-vitro studies, derived from the Latin term "in glass," involve experiments performed in controlled laboratory settings using cells or tissues. It is important to note that these products are not categorized as medicines or drugs, and they have not received approval from the FDA for the prevention, treatment, or cure of any medical condition, ailment, or disease. We must emphasize that any form of bodily introduction of these products into humans or animals is strictly prohibited by law. It is essential to adhere to these guidelines to ensure compliance with legal and ethical standards in research and experimentation.