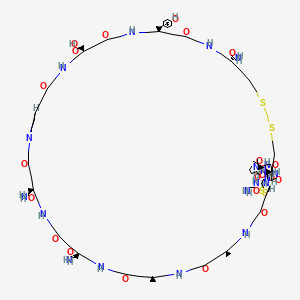
alpha-conotoxin PnIA
Overview
Description
Alpha-conotoxin PnIA is a peptide neurotoxin isolated from the venom of the marine cone snail species, Conus pennaceus. This compound is part of the alpha-conotoxin family, which is known for its ability to selectively target and block nicotinic acetylcholine receptors (nAChRs) in the nervous system . This compound specifically targets neuronal nAChRs, distinguishing it from other alpha-conotoxins that may target skeletal muscle subtypes .
Mechanism of Action
Target of Action
Alpha-conotoxin PnIA primarily targets the nicotinic acetylcholine receptors (nAChRs), which are found both in the neuromuscular junction and central nervous system . These receptors play a crucial role in transmitting signals in the nervous system. This compound is a selective antagonist of α3β2 nAChR receptors .
Mode of Action
This compound interacts with its targets, the nAChRs, by binding to a small pocket situated above the β9/β10 hairpin of the (+) α7 nAChR . This interaction blocks the receptors, preventing the transmission of signals . The structure of PnIA is characterized by distinct hydrophobic and polar surfaces, a separation of the sole positive and negative charges, a hydrophobic region, and a protruding tyrosine side chain . These features may be important for the specific interaction of PnIA with neuronal nAChR .
Biochemical Pathways
The action of this compound affects the biochemical pathways associated with the function of nAChRs. By blocking these receptors, it disrupts the normal flow of signals in the nervous system . The exact downstream effects of this disruption can vary depending on the specific subtype of nAChR that is targeted and the location of these receptors in the body.
Pharmacokinetics
The compact architecture of the pnia toxin provides a rigid framework for presentation of chemical groups that are required for activity . This structure, along with its distinct hydrophobic and polar surfaces, may influence its bioavailability .
Result of Action
The primary result of this compound’s action is the inhibition of nAChRs, which prevents the transmission of signals in the nervous system . This can have various effects at the molecular and cellular level, depending on the specific subtype of nAChR that is targeted. For example, it has been shown that alpha-conotoxins and alpha-cobratoxin can promote the proliferation of C6 glioma cells .
Biochemical Analysis
Biochemical Properties
Alpha-Conotoxin PnIA blocks mammalian alpha-3-beta-2/CHRNA3-CHRNB2 and alpha-7/CHRNA7 nAChRs . It interacts with a hydrophobic pocket between two acetylcholine receptor subunits . The 16-residue peptides PnIA and PnIB from Conus pennaceus incorporate the same disulfide framework as other alpha-conotoxins .
Cellular Effects
This compound exhibits low cytotoxicity but enhances the antitumor effect of certain inhibitors . For instance, it enhances the antitumor effect of baicalein 1.4-fold after 24 hours and that of nordihydroguaiaretic acid 1.8–3.9-fold after 48 hours of cell cultivation .
Molecular Mechanism
The crystal structure of synthetic this compound incorporates a beta turn followed by two alpha-helical turns . The conformation is stabilized by two disulfide bridges that form the interior of the molecule, with all other side chains oriented outwards . These features may be important for the specific interaction of this compound with neuronal nAChR .
Temporal Effects in Laboratory Settings
The effects of this compound vary over time. For instance, it enhances the antitumor effect of baicalein 1.4-fold after 24 hours and that of nordihydroguaiaretic acid 1.8–3.9-fold after 48 hours of cell cultivation .
Dosage Effects in Animal Models
In vivo, baicalein, Alpha-Conotoxins MII and PnIA inhibit Ehrlich carcinoma growth and increase mouse survival . These effects are greatly enhanced by the combined application of Alpha-Conotoxin MII with indomethacin or Conotoxin PnIA with baicalein .
Preparation Methods
Synthetic Routes and Reaction Conditions: Alpha-conotoxin PnIA can be synthesized using solid-phase peptide synthesis (SPPS). This method involves the sequential addition of protected amino acids to a growing peptide chain anchored to a solid resin. The synthesis is typically carried out under mildly basic conditions to facilitate the coupling reactions. The disulfide bridges, which are crucial for the structural integrity of the peptide, are formed through oxidative folding .
Industrial Production Methods: Industrial production of this compound involves large-scale SPPS, followed by purification using high-performance liquid chromatography (HPLC). The peptide is then subjected to oxidative folding to ensure the correct disulfide bridge formation. The final product is characterized using techniques such as mass spectrometry and nuclear magnetic resonance (NMR) spectroscopy to confirm its structure and purity .
Chemical Reactions Analysis
Types of Reactions: Alpha-conotoxin PnIA primarily undergoes oxidation reactions to form its disulfide bridges. These reactions are essential for the peptide to attain its biologically active conformation .
Common Reagents and Conditions: The oxidative folding of this compound is typically carried out in an aqueous buffer containing oxidizing agents such as hydrogen peroxide or iodine. The reaction conditions are carefully controlled to ensure the formation of the correct disulfide bridges without over-oxidation .
Major Products: The major product of these reactions is the correctly folded this compound with its native disulfide bridge pattern. Incorrectly folded isomers may also form but are typically separated and discarded during the purification process .
Scientific Research Applications
Alpha-conotoxin PnIA has a wide range of scientific research applications:
Chemistry: It serves as a model compound for studying peptide synthesis and folding mechanisms.
Industry: It is utilized in the development of novel pharmacological probes and drug leads targeting nAChRs.
Comparison with Similar Compounds
Alpha-conotoxin PnIA is unique among alpha-conotoxins due to its specific targeting of neuronal nAChRs rather than skeletal muscle subtypes. Similar compounds include:
Alpha-conotoxin PnIB: Another peptide from Conus pennaceus, which also targets neuronal nAChRs but with different subtype selectivity.
Alpha-conotoxin MII: Isolated from Conus magus, this peptide targets the alpha3beta2 nAChR subtype but differs in its amino acid sequence and disulfide bridge pattern.
Alpha-conotoxin ImI: From Conus imperialis, this peptide targets the alpha7 nAChR subtype and is used as a pharmacological tool to study this receptor.
This compound’s unique sequence and disulfide bridge pattern contribute to its specific receptor interactions and biological activity, making it a valuable tool in neuroscience research .
Properties
IUPAC Name |
2-[(1R,6R,9S,12S,15S,21S,24S,27S,30S,33R,36S,42S,48S,51S,56R)-56-[(2-aminoacetyl)amino]-21,24-bis(2-amino-2-oxoethyl)-6-carbamoyl-51-(hydroxymethyl)-9-[(4-hydroxyphenyl)methyl]-27,30-dimethyl-48-(2-methylpropyl)-8,11,14,20,23,26,29,32,35,41,47,50,53,55-tetradecaoxo-3,4,58,59-tetrathia-7,10,13,19,22,25,28,31,34,40,46,49,52,54-tetradecazapentacyclo[31.20.7.015,19.036,40.042,46]hexacontan-12-yl]acetic acid | |
---|---|---|
Source | PubChem | |
URL | https://pubchem.ncbi.nlm.nih.gov | |
Description | Data deposited in or computed by PubChem | |
InChI |
InChI=1S/C65H95N19O22S4/c1-29(2)18-37-63(104)84-17-7-10-46(84)65(106)83-16-6-9-45(83)62(103)81-42-27-110-108-26-41(72-49(89)23-66)59(100)80-43(60(101)78-39(24-85)57(98)76-37)28-109-107-25-40(51(69)92)79-54(95)34(19-32-11-13-33(86)14-12-32)74-56(97)36(22-50(90)91)75-61(102)44-8-5-15-82(44)64(105)38(21-48(68)88)77-55(96)35(20-47(67)87)73-53(94)31(4)70-52(93)30(3)71-58(42)99/h11-14,29-31,34-46,85-86H,5-10,15-28,66H2,1-4H3,(H2,67,87)(H2,68,88)(H2,69,92)(H,70,93)(H,71,99)(H,72,89)(H,73,94)(H,74,97)(H,75,102)(H,76,98)(H,77,96)(H,78,101)(H,79,95)(H,80,100)(H,81,103)(H,90,91)/t30-,31-,34-,35-,36-,37-,38-,39-,40-,41-,42-,43-,44-,45-,46-/m0/s1 | |
Source | PubChem | |
URL | https://pubchem.ncbi.nlm.nih.gov | |
Description | Data deposited in or computed by PubChem | |
InChI Key |
VUVGEYBNLLGGBG-MVPSLEAZSA-N | |
Source | PubChem | |
URL | https://pubchem.ncbi.nlm.nih.gov | |
Description | Data deposited in or computed by PubChem | |
Canonical SMILES |
CC1C(=O)NC(C(=O)NC(C(=O)N2CCCC2C(=O)NC(C(=O)NC(C(=O)NC(CSSCC3C(=O)NC(C(=O)NC(C(=O)N4CCCC4C(=O)N5CCCC5C(=O)NC(CSSCC(C(=O)N3)NC(=O)CN)C(=O)NC(C(=O)N1)C)CC(C)C)CO)C(=O)N)CC6=CC=C(C=C6)O)CC(=O)O)CC(=O)N)CC(=O)N | |
Source | PubChem | |
URL | https://pubchem.ncbi.nlm.nih.gov | |
Description | Data deposited in or computed by PubChem | |
Isomeric SMILES |
C[C@H]1C(=O)N[C@H](C(=O)N[C@H](C(=O)N2CCC[C@H]2C(=O)N[C@H](C(=O)N[C@H](C(=O)N[C@@H](CSSC[C@H]3C(=O)N[C@H](C(=O)N[C@H](C(=O)N4CCC[C@H]4C(=O)N5CCC[C@H]5C(=O)N[C@@H](CSSC[C@@H](C(=O)N3)NC(=O)CN)C(=O)N[C@H](C(=O)N1)C)CC(C)C)CO)C(=O)N)CC6=CC=C(C=C6)O)CC(=O)O)CC(=O)N)CC(=O)N | |
Source | PubChem | |
URL | https://pubchem.ncbi.nlm.nih.gov | |
Description | Data deposited in or computed by PubChem | |
Molecular Formula |
C65H95N19O22S4 | |
Source | PubChem | |
URL | https://pubchem.ncbi.nlm.nih.gov | |
Description | Data deposited in or computed by PubChem | |
Molecular Weight |
1622.8 g/mol | |
Source | PubChem | |
URL | https://pubchem.ncbi.nlm.nih.gov | |
Description | Data deposited in or computed by PubChem | |
CAS No. |
705300-84-1 | |
Record name | n/a | |
Source | European Chemicals Agency (ECHA) | |
URL | https://echa.europa.eu/information-on-chemicals | |
Description | The European Chemicals Agency (ECHA) is an agency of the European Union which is the driving force among regulatory authorities in implementing the EU's groundbreaking chemicals legislation for the benefit of human health and the environment as well as for innovation and competitiveness. | |
Explanation | Use of the information, documents and data from the ECHA website is subject to the terms and conditions of this Legal Notice, and subject to other binding limitations provided for under applicable law, the information, documents and data made available on the ECHA website may be reproduced, distributed and/or used, totally or in part, for non-commercial purposes provided that ECHA is acknowledged as the source: "Source: European Chemicals Agency, http://echa.europa.eu/". Such acknowledgement must be included in each copy of the material. ECHA permits and encourages organisations and individuals to create links to the ECHA website under the following cumulative conditions: Links can only be made to webpages that provide a link to the Legal Notice page. | |
Q1: What is the primary target of α-conotoxin PnIA and how does it exert its effect?
A1: α-Conotoxin PnIA is a potent antagonist of neuronal nicotinic acetylcholine receptors (nAChRs) [, , , , , , , , ]. By binding to these receptors, it prevents the binding of acetylcholine, the endogenous neurotransmitter, ultimately inhibiting the normal function of these ion channels [, , ].
Q2: Which specific nAChR subtype does α-conotoxin PnIA show preference for?
A2: While α-conotoxin PnIA can inhibit various nAChR subtypes, it exhibits a higher affinity for the α3β2 subtype compared to the α7 subtype [, , ]. This selectivity makes it a valuable tool for studying the roles of different nAChR subtypes in neuronal signaling.
Q3: How does the structure of α-conotoxin PnIA contribute to its activity and selectivity?
A3: α-Conotoxin PnIA adopts a compact structure stabilized by two disulfide bridges, forming a core scaffold observed in other neuronal α-conotoxins [, , ]. This scaffold supports two loops that present crucial amino acid side chains for interaction with nAChRs. Notably, residue 10 plays a significant role in determining subtype selectivity. For example, substituting Alanine with Leucine at position 10 ([A10L]PnIA) increases its affinity for the α7 subtype [, ].
Q4: What techniques are employed to study the structure and interaction of α-conotoxin PnIA with nAChRs?
A4: Researchers utilize a combination of techniques to elucidate the structural features and binding mechanism of α-conotoxin PnIA. Nuclear Magnetic Resonance (NMR) spectroscopy has been instrumental in determining its solution structure, revealing the presence of β-turns and a 310 helix [, ]. X-ray crystallography provides high-resolution structures, highlighting the conserved disulfide framework [, ]. Furthermore, studies involving chimeric nAChR subunits and point mutations help pinpoint the specific amino acid residues crucial for toxin binding and subtype selectivity [].
Q5: Has α-conotoxin PnIA been used to investigate the functional properties of nAChRs?
A5: Yes, α-conotoxin PnIA has been instrumental in dissecting the components of the nicotinic acetylcholine-induced current in mammalian parasympathetic neurons []. It has also been used in studies to understand the different states of the α7 nAChR, particularly in the context of the α7-L247T mutation, which exhibits altered desensitization properties [].
Disclaimer and Information on In-Vitro Research Products
Please be aware that all articles and product information presented on BenchChem are intended solely for informational purposes. The products available for purchase on BenchChem are specifically designed for in-vitro studies, which are conducted outside of living organisms. In-vitro studies, derived from the Latin term "in glass," involve experiments performed in controlled laboratory settings using cells or tissues. It is important to note that these products are not categorized as medicines or drugs, and they have not received approval from the FDA for the prevention, treatment, or cure of any medical condition, ailment, or disease. We must emphasize that any form of bodily introduction of these products into humans or animals is strictly prohibited by law. It is essential to adhere to these guidelines to ensure compliance with legal and ethical standards in research and experimentation.