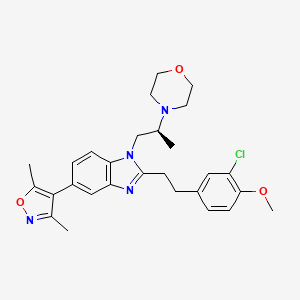
Sgc-cbp30
- Click on QUICK INQUIRY to receive a quote from our team of experts.
- With the quality product at a COMPETITIVE price, you can focus more on your research.
Overview
Description
SGC-CBP30 is a potent and selective inhibitor of the bromodomains of CREB-binding protein (CBP) and p300. These proteins are histone acetyltransferases that play a crucial role in regulating gene expression by acting as transcriptional co-activators. The compound has shown significant potential in scientific research, particularly in the fields of cancer and immunology .
Mechanism of Action
Target of Action
SGC-CBP30 is a potent and highly selective inhibitor of the bromodomains of CREB-binding protein (CBP) and p300 . These proteins are histone acetyltransferases that can act as transcriptional co-activators to regulate gene expression . Mutations in CBP and p300 have been associated with cancer .
Mode of Action
This compound interacts with the bromodomains of CBP and p300, inhibiting their function . The bromodomains of these proteins facilitate acetylation of lysine residues . By inhibiting CBP and p300, this compound impacts gene expression and cellular processes .
Biochemical Pathways
The inhibition of CBP and p300 by this compound affects various biochemical pathways. CBP and p300 are involved in many biological processes like maintenance of genomic stability by affecting DNA replication and DNA repair as well as cell growth, transformation, and development . They also play an essential role in neuronal plasticity/memory formation, hematopoiesis, and energy homeostasis .
Pharmacokinetics
It’s known that the compound is soluble in dmso at least up to 50mm , which suggests it may have good bioavailability.
Result of Action
The inhibition of CBP and p300 by this compound has several molecular and cellular effects. It has been shown to reduce inflammatory cell infiltration in the lungs, liver, colon, and kidney of a septic mouse model . In cancer research, it has been used to inhibit cell proliferation and induce apoptosis by downregulating uridine phosphorylase 1 expression in human lung adenocarcinoma cells . It can also diminish the viability of human pancreatic cancer cells when used in combination with the bromodomain and extra-terminal domain (BET) inhibitors .
Action Environment
The action of this compound can be influenced by various environmental factors. For instance, the compound’s efficacy can be affected by the presence of other molecules in the cellular environment. As an example, it has been shown to have a synergistic effect with BET inhibitors in reducing the viability of pancreatic cancer cells . .
Biochemical Analysis
Biochemical Properties
Sgc-cbp30 plays a significant role in biochemical reactions, particularly as an inhibitor of CREBBP and EP300 . These proteins are histone acetyltransferases that can act as transcriptional co-activators to regulate gene expression . The bromodomain of these proteins facilitates acetylation of lysine residues .
Cellular Effects
This compound impacts various types of cells and cellular processes. For instance, it has been used to reduce inflammatory cell infiltration in the lungs, liver, colon, and kidney of a septic mouse model . It also inhibits cell proliferation and induces apoptosis by downregulating uridine phosphorylase 1 expression in human lung adenocarcinoma cells .
Molecular Mechanism
At the molecular level, this compound exerts its effects through binding interactions with biomolecules, specifically the bromodomains of CBP and EP300 . This interaction inhibits the acetylation activity of these proteins, thereby influencing gene expression .
Metabolic Pathways
This compound is involved in metabolic pathways related to gene expression regulation. It interacts with the enzymes CBP and EP300, influencing the acetylation of lysine residues .
Preparation Methods
The synthesis of SGC-CBP30 involves several steps, starting with the preparation of the core benzimidazole structure. The synthetic route typically includes the following steps:
Formation of the Benzimidazole Core: This involves the reaction of o-phenylenediamine with a carboxylic acid derivative under acidic conditions.
Introduction of Substituents: The benzimidazole core is then functionalized with various substituents, including a 3-chloro-4-methoxyphenyl group and a 3,5-dimethyl-4-isoxazolyl group.
Final Coupling: The final step involves coupling the functionalized benzimidazole with a morpholine derivative to yield this compound
Chemical Reactions Analysis
SGC-CBP30 undergoes several types of chemical reactions:
Oxidation: The compound can be oxidized under specific conditions to form various oxidized derivatives.
Reduction: Reduction reactions can be performed to modify the functional groups on the benzimidazole core.
Substitution: Substitution reactions are commonly used to introduce different substituents onto the benzimidazole core or the isoxazole ring.
Common Reagents and Conditions: Typical reagents include oxidizing agents like potassium permanganate, reducing agents like sodium borohydride, and various nucleophiles for substitution reactions
Scientific Research Applications
SGC-CBP30 has a wide range of applications in scientific research:
Cancer Research: It has been used to inhibit cell proliferation and induce apoptosis in various cancer cell lines, including lung adenocarcinoma and pancreatic cancer cells.
Immunology: The compound has shown anti-inflammatory effects by reducing the secretion of interleukin-17A in T helper 17 cells.
Gene Regulation: This compound has been used to study the regulation of histone acetyltransferase activity and its impact on gene expression.
Other Applications:
Comparison with Similar Compounds
SGC-CBP30 is unique in its high selectivity for the bromodomains of CBP and p300. Similar compounds include:
I-CBP112: Another selective inhibitor of CBP/p300 bromodomains, but with different selectivity and potency profiles.
GNE-049: A selective inhibitor of the bromodomain and extra-terminal (BET) family of proteins, which also includes CBP and p300.
JQ1: A well-known BET inhibitor that targets a broader range of bromodomains compared to this compound
This compound stands out due to its high selectivity and potency, making it a valuable tool in studying the specific roles of CBP and p300 in various biological processes .
Biological Activity
SGC-CBP30 is a selective inhibitor of the bromodomains of the transcriptional coactivators CBP (CREB-binding protein) and p300, which play critical roles in various cellular processes including gene expression, cell cycle regulation, and inflammation. This article provides a comprehensive overview of the biological activity of this compound, highlighting its mechanisms of action, effects in various cellular contexts, and implications for therapeutic applications.
- Chemical Name : 2-[2-(3-Chloro-4-methoxyphenyl)ethyl]-5-(dimethyl-1,2-oxazol-4-yl)-1-[(2S)-2-(morpholin-4-yl)propyl]-1H-1,3-benzodiazole
- Molecular Weight : 509 g/mol
- Purity : ≥98%
- Kd Values : 21 nM (CBP), 32 nM (p300)
This compound functions by selectively inhibiting the bromodomains of CBP and p300, leading to reduced histone acetylation and altered transcriptional regulation. The compound exhibits significant selectivity for CBP over BRD4, with a selectivity ratio of 40-fold for BRD4(1) and 250-fold for BRD4(2) . This selectivity is crucial for minimizing off-target effects in therapeutic applications.
Inhibition of α-Synuclein Aggregation
In studies involving primary mouse embryonic dopaminergic neurons, this compound demonstrated a capacity to reduce α-synuclein aggregation, a hallmark of neurodegenerative diseases such as Parkinson's disease. At concentrations as low as 1 μM, this compound significantly decreased PFF-induced α-synuclein aggregation, with statistical significance established through two-way ANOVA (p < 0.0001) . However, at higher concentrations (10 μM), toxicity to TH-positive cells was observed, although this effect was mitigated when combined with PFF treatment .
Impact on Cancer Cell Lines
This compound has been shown to induce cell cycle arrest and apoptosis in CBP-deficient cancer cells. In lung cancer models with deleterious CBP mutations, treatment with this compound led to a significant reduction in cell viability and induced synthetic lethality linked to MYC downregulation . Additionally, it displayed preferential activity against multiple myeloma cell lines compared to leukemic lines, suggesting its potential specificity in targeting certain cancer types .
Anti-inflammatory Properties
Research indicates that this compound can inhibit HMGB1-mediated inflammatory responses. In vitro studies demonstrated that the compound effectively suppressed LPS-induced expression and release of HMGB1 and attenuated its pro-inflammatory activity by reactivating protein phosphatase 2A (PP2A) and stabilizing MAPK phosphatase 1 (MKP-1) . This suggests its potential utility in treating inflammation-related conditions.
Case Studies
Study | Cell Type | Concentration | Effect | Significance |
---|---|---|---|---|
Study 1 | Dopaminergic Neurons | 1 μM | Decreased α-synuclein aggregation | p < 0.0001 |
Study 2 | Lung Cancer Cells | Varies | Induced apoptosis in CBP-deficient cells | Synthetic lethality observed |
Study 3 | Chordoma Cells | Varies | Suppressed proliferation when combined with cisplatin | Significant reduction in clonogenic ability |
Properties
IUPAC Name |
4-[(2S)-1-[2-[2-(3-chloro-4-methoxyphenyl)ethyl]-5-(3,5-dimethyl-1,2-oxazol-4-yl)benzimidazol-1-yl]propan-2-yl]morpholine |
Source
|
---|---|---|
Source | PubChem | |
URL | https://pubchem.ncbi.nlm.nih.gov | |
Description | Data deposited in or computed by PubChem | |
InChI |
InChI=1S/C28H33ClN4O3/c1-18(32-11-13-35-14-12-32)17-33-25-8-7-22(28-19(2)31-36-20(28)3)16-24(25)30-27(33)10-6-21-5-9-26(34-4)23(29)15-21/h5,7-9,15-16,18H,6,10-14,17H2,1-4H3/t18-/m0/s1 |
Source
|
Source | PubChem | |
URL | https://pubchem.ncbi.nlm.nih.gov | |
Description | Data deposited in or computed by PubChem | |
InChI Key |
GEPYBHCJBORHCE-SFHVURJKSA-N |
Source
|
Source | PubChem | |
URL | https://pubchem.ncbi.nlm.nih.gov | |
Description | Data deposited in or computed by PubChem | |
Canonical SMILES |
CC1=C(C(=NO1)C)C2=CC3=C(C=C2)N(C(=N3)CCC4=CC(=C(C=C4)OC)Cl)CC(C)N5CCOCC5 |
Source
|
Source | PubChem | |
URL | https://pubchem.ncbi.nlm.nih.gov | |
Description | Data deposited in or computed by PubChem | |
Isomeric SMILES |
CC1=C(C(=NO1)C)C2=CC3=C(C=C2)N(C(=N3)CCC4=CC(=C(C=C4)OC)Cl)C[C@H](C)N5CCOCC5 |
Source
|
Source | PubChem | |
URL | https://pubchem.ncbi.nlm.nih.gov | |
Description | Data deposited in or computed by PubChem | |
Molecular Formula |
C28H33ClN4O3 |
Source
|
Source | PubChem | |
URL | https://pubchem.ncbi.nlm.nih.gov | |
Description | Data deposited in or computed by PubChem | |
Molecular Weight |
509.0 g/mol |
Source
|
Source | PubChem | |
URL | https://pubchem.ncbi.nlm.nih.gov | |
Description | Data deposited in or computed by PubChem | |
Disclaimer and Information on In-Vitro Research Products
Please be aware that all articles and product information presented on BenchChem are intended solely for informational purposes. The products available for purchase on BenchChem are specifically designed for in-vitro studies, which are conducted outside of living organisms. In-vitro studies, derived from the Latin term "in glass," involve experiments performed in controlled laboratory settings using cells or tissues. It is important to note that these products are not categorized as medicines or drugs, and they have not received approval from the FDA for the prevention, treatment, or cure of any medical condition, ailment, or disease. We must emphasize that any form of bodily introduction of these products into humans or animals is strictly prohibited by law. It is essential to adhere to these guidelines to ensure compliance with legal and ethical standards in research and experimentation.