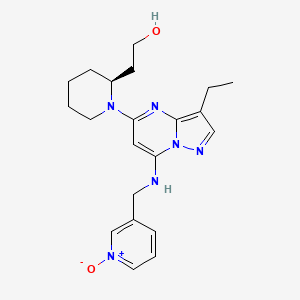
Dinaciclib
Overview
Description
Dinaciclib is a potent small molecule inhibitor of cyclin-dependent kinases (CDKs), specifically targeting CDK1, CDK2, CDK5, and CDK9 . It has shown promising results in various preclinical and clinical studies, particularly in the treatment of cancers such as multiple myeloma, cholangiocarcinoma, and chronic lymphocytic leukemia . By inhibiting these kinases, this compound disrupts cell cycle progression and transcriptional processes, leading to the induction of apoptosis in cancer cells .
Scientific Research Applications
Dinaciclib has a wide range of scientific research applications, including:
Chemistry: Used as a tool compound to study the role of CDKs in various biochemical pathways.
Biology: Investigated for its effects on cell cycle regulation, apoptosis, and transcriptional control.
Medicine: Explored as a therapeutic agent for various cancers, including multiple myeloma, cholangiocarcinoma, and chronic lymphocytic leukemia
Mechanism of Action
Target of Action
Dinaciclib is a small molecule multi-cyclin-dependent kinase (CDK) inhibitor . It primarily targets CDK2, CDK5, and CDK9 . These CDKs play crucial roles in regulating cell cycle progression and transcriptional processes, making them attractive therapeutic targets for cancer .
Mode of Action
This compound interacts with its primary targets (CDK2, CDK5, and CDK9) by suppressing their activity . This suppression leads to a decrease in the phosphorylation of the retinoblastoma (Rb) tumor suppressor protein, which is a key regulator of cell cycle progression . Additionally, this compound has been shown to interact with the acetyl-lysine recognition site of bromodomains .
Biochemical Pathways
This compound affects multiple biochemical pathways. It inhibits the canonical/β-catenin dependent Wnt signaling pathway, a known resistance mechanism to bromo- and extra-terminal domain (BET) inhibitors in acute myeloid leukemia (AML) . This compound suppresses Wnt signaling at multiple levels, including downregulation of β-catenin, the Wnt co-receptor LRP6, as well as many Wnt pathway components and targets .
Result of Action
This compound has been shown to significantly suppress cell proliferation and induce apoptotic activity in various cell lines . It achieves this by suppressing the expression of its molecular targets (CDK2/5/9) and anti-apoptotic proteins BCL-XL and BCL2 . In addition, this compound has been shown to induce caspase 3/7 activity, which plays a crucial role in the execution-phase of cell apoptosis .
Safety and Hazards
Future Directions
Dinaciclib is currently being evaluated in clinical trials for various cancer indications . It has shown promising results in early phase clinical trials for additional breast cancer subtypes and non-breast malignancies . Further clinical-translational research is needed to advance patient selection and combinatorial treatment strategies with this compound in breast cancer and other malignancies .
Biochemical Analysis
Biochemical Properties
Dinaciclib interacts with cyclin-dependent kinases (CDKs), which are crucial regulators of cell cycle progression and transcriptional processes . It specifically targets CDK2, CDK5, and CDK9 . The nature of these interactions involves the inhibition of these kinases, thereby disrupting cell cycle progression and transcriptional processes .
Cellular Effects
This compound has significant effects on various types of cells and cellular processes. It suppresses cell proliferation and induces apoptotic activity in patient-derived xenograft cells and cholangiocarcinoma cell lines . This compound also influences cell function by suppressing the expression of its molecular targets CDK2/5/9, and anti-apoptotic BCL-XL and BCL2 proteins .
Molecular Mechanism
This compound exerts its effects at the molecular level by inhibiting cyclin-dependent kinases (CDKs). This inhibition leads to the suppression of cell cycle progression and transcriptional processes . This compound also suppresses the expression of its molecular targets CDK2/5/9, and anti-apoptotic BCL-XL and BCL2 proteins .
Temporal Effects in Laboratory Settings
This compound has shown to produce a robust and sustained inhibition of tumor progression in vivo in a patient-derived xenograft mouse model . This suggests that this compound has long-term effects on cellular function. More studies are needed to understand its stability and degradation over time.
Dosage Effects in Animal Models
The effects of this compound vary with different dosages in animal models. For instance, this compound administered at a dose of 30 to 50 mg/m² in a 21-day cycle has shown to suppress tumor progression in a patient-derived xenograft mouse model
Metabolic Pathways
It is known that this compound inhibits CDKs, which play crucial roles in cell cycle progression and transcriptional processes . This could potentially affect metabolic flux or metabolite levels.
Preparation Methods
Synthetic Routes and Reaction Conditions: The synthesis of dinaciclib involves multiple steps, starting with the formation of the pyrazolo[1,5-a]pyrimidine core. This is followed by the introduction of various substituents to achieve the final structure. The key steps include:
Formation of the pyrazolo[1,5-a]pyrimidine core: This involves the cyclization of appropriate precursors under controlled conditions.
Introduction of substituents: Various substituents are introduced through nucleophilic substitution, reduction, and other organic reactions.
Industrial Production Methods: Industrial production of this compound follows similar synthetic routes but is optimized for large-scale production. This includes the use of high-yield reactions, efficient purification techniques, and stringent quality control measures to ensure the purity and efficacy of the final product .
Chemical Reactions Analysis
Types of Reactions: Dinaciclib undergoes several types of chemical reactions, including:
Oxidation: this compound can be oxidized under specific conditions to form various oxidized derivatives.
Reduction: Reduction reactions can modify certain functional groups within the molecule.
Substitution: Nucleophilic and electrophilic substitution reactions are common in the synthesis and modification of this compound.
Oxidation: Common oxidizing agents include hydrogen peroxide and potassium permanganate.
Reduction: Reducing agents such as sodium borohydride and lithium aluminum hydride are used.
Substitution: Various nucleophiles and electrophiles are employed depending on the desired modification.
Major Products: The major products formed from these reactions include various derivatives of this compound, which may have different biological activities and properties .
Comparison with Similar Compounds
Similar Compounds:
Flavopiridol: Another CDK inhibitor with a broader target profile but associated with higher toxicity.
Palbociclib: Selectively inhibits CDK4 and CDK6, used primarily in breast cancer treatment.
Ribociclib: Similar to palbociclib, targets CDK4 and CDK6.
Uniqueness of Dinaciclib: this compound’s unique ability to target multiple CDKs (CDK1, CDK2, CDK5, and CDK9) sets it apart from other CDK inhibitors. This broad-spectrum inhibition allows for more effective disruption of cancer cell proliferation and survival pathways . Additionally, this compound has shown promising results in combination therapies, enhancing the efficacy of other anticancer agents .
Properties
IUPAC Name |
2-[(2S)-1-[3-ethyl-7-[(1-oxidopyridin-1-ium-3-yl)methylamino]pyrazolo[1,5-a]pyrimidin-5-yl]piperidin-2-yl]ethanol | |
---|---|---|
Source | PubChem | |
URL | https://pubchem.ncbi.nlm.nih.gov | |
Description | Data deposited in or computed by PubChem | |
InChI |
InChI=1S/C21H28N6O2/c1-2-17-14-23-27-19(22-13-16-6-5-9-25(29)15-16)12-20(24-21(17)27)26-10-4-3-7-18(26)8-11-28/h5-6,9,12,14-15,18,22,28H,2-4,7-8,10-11,13H2,1H3/t18-/m0/s1 | |
Source | PubChem | |
URL | https://pubchem.ncbi.nlm.nih.gov | |
Description | Data deposited in or computed by PubChem | |
InChI Key |
PIMQWRZWLQKKBJ-SFHVURJKSA-N | |
Source | PubChem | |
URL | https://pubchem.ncbi.nlm.nih.gov | |
Description | Data deposited in or computed by PubChem | |
Canonical SMILES |
CCC1=C2N=C(C=C(N2N=C1)NCC3=C[N+](=CC=C3)[O-])N4CCCCC4CCO | |
Source | PubChem | |
URL | https://pubchem.ncbi.nlm.nih.gov | |
Description | Data deposited in or computed by PubChem | |
Isomeric SMILES |
CCC1=C2N=C(C=C(N2N=C1)NCC3=C[N+](=CC=C3)[O-])N4CCCC[C@H]4CCO | |
Source | PubChem | |
URL | https://pubchem.ncbi.nlm.nih.gov | |
Description | Data deposited in or computed by PubChem | |
Molecular Formula |
C21H28N6O2 | |
Source | PubChem | |
URL | https://pubchem.ncbi.nlm.nih.gov | |
Description | Data deposited in or computed by PubChem | |
Molecular Weight |
396.5 g/mol | |
Source | PubChem | |
URL | https://pubchem.ncbi.nlm.nih.gov | |
Description | Data deposited in or computed by PubChem | |
CAS No. |
779353-01-4 | |
Record name | Dinaciclib | |
Source | CAS Common Chemistry | |
URL | https://commonchemistry.cas.org/detail?cas_rn=779353-01-4 | |
Description | CAS Common Chemistry is an open community resource for accessing chemical information. Nearly 500,000 chemical substances from CAS REGISTRY cover areas of community interest, including common and frequently regulated chemicals, and those relevant to high school and undergraduate chemistry classes. This chemical information, curated by our expert scientists, is provided in alignment with our mission as a division of the American Chemical Society. | |
Explanation | The data from CAS Common Chemistry is provided under a CC-BY-NC 4.0 license, unless otherwise stated. | |
Record name | Dinaciclib | |
Source | DrugBank | |
URL | https://www.drugbank.ca/drugs/DB12021 | |
Description | The DrugBank database is a unique bioinformatics and cheminformatics resource that combines detailed drug (i.e. chemical, pharmacological and pharmaceutical) data with comprehensive drug target (i.e. sequence, structure, and pathway) information. | |
Explanation | Creative Common's Attribution-NonCommercial 4.0 International License (http://creativecommons.org/licenses/by-nc/4.0/legalcode) | |
Record name | (S)-3-((3-ethyl-5-(2-(2-hydroxyethyl)piperidin-1-yl)pyrazolo[1,5-a]pyrimidin-7-ylamino)methyl)pyridine 1-oxide | |
Source | European Chemicals Agency (ECHA) | |
URL | https://echa.europa.eu/information-on-chemicals | |
Description | The European Chemicals Agency (ECHA) is an agency of the European Union which is the driving force among regulatory authorities in implementing the EU's groundbreaking chemicals legislation for the benefit of human health and the environment as well as for innovation and competitiveness. | |
Explanation | Use of the information, documents and data from the ECHA website is subject to the terms and conditions of this Legal Notice, and subject to other binding limitations provided for under applicable law, the information, documents and data made available on the ECHA website may be reproduced, distributed and/or used, totally or in part, for non-commercial purposes provided that ECHA is acknowledged as the source: "Source: European Chemicals Agency, http://echa.europa.eu/". Such acknowledgement must be included in each copy of the material. ECHA permits and encourages organisations and individuals to create links to the ECHA website under the following cumulative conditions: Links can only be made to webpages that provide a link to the Legal Notice page. | |
Record name | DINACICLIB | |
Source | FDA Global Substance Registration System (GSRS) | |
URL | https://gsrs.ncats.nih.gov/ginas/app/beta/substances/4V8ECV0NBQ | |
Description | The FDA Global Substance Registration System (GSRS) enables the efficient and accurate exchange of information on what substances are in regulated products. Instead of relying on names, which vary across regulatory domains, countries, and regions, the GSRS knowledge base makes it possible for substances to be defined by standardized, scientific descriptions. | |
Explanation | Unless otherwise noted, the contents of the FDA website (www.fda.gov), both text and graphics, are not copyrighted. They are in the public domain and may be republished, reprinted and otherwise used freely by anyone without the need to obtain permission from FDA. Credit to the U.S. Food and Drug Administration as the source is appreciated but not required. | |
Disclaimer and Information on In-Vitro Research Products
Please be aware that all articles and product information presented on BenchChem are intended solely for informational purposes. The products available for purchase on BenchChem are specifically designed for in-vitro studies, which are conducted outside of living organisms. In-vitro studies, derived from the Latin term "in glass," involve experiments performed in controlled laboratory settings using cells or tissues. It is important to note that these products are not categorized as medicines or drugs, and they have not received approval from the FDA for the prevention, treatment, or cure of any medical condition, ailment, or disease. We must emphasize that any form of bodily introduction of these products into humans or animals is strictly prohibited by law. It is essential to adhere to these guidelines to ensure compliance with legal and ethical standards in research and experimentation.