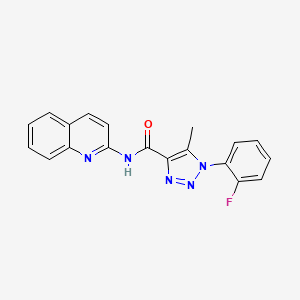
1-(2-fluorophenyl)-5-methyl-N-quinolin-2-yltriazole-4-carboxamide
Overview
Description
YW1159, formally known as 1-(2-fluorophenyl)-5-methyl-N-2-quinolinyl-1H-1,2,3-triazole-4-carboxamide, is a chemical compound that acts as an inhibitor of the Wnt signaling pathway. This pathway is crucial in various biological processes, including cell proliferation, differentiation, and migration. The inhibition of Wnt signaling by YW1159 has significant implications in the treatment of diseases such as cancer and metabolic disorders .
Scientific Research Applications
YW1159 has a wide range of scientific research applications, including:
Chemistry: Used as a tool compound to study the Wnt signaling pathway and its inhibition.
Biology: Investigated for its effects on cell proliferation, differentiation, and migration.
Medicine: Explored as a potential therapeutic agent for treating cancers and metabolic disorders.
Industry: Utilized in the development of new drugs and therapeutic agents targeting the Wnt signaling pathway
Mechanism of Action
YW1159 exerts its effects by inhibiting the Wnt signaling pathway. The compound binds to specific molecular targets within the pathway, preventing the activation of downstream signaling components. This inhibition leads to a decrease in cell proliferation and an increase in cell differentiation and apoptosis. The molecular targets and pathways involved include β-catenin and other key proteins in the Wnt signaling cascade .
Similar Compounds:
XAV939: Another inhibitor of the Wnt signaling pathway, but with a different mechanism of action.
ICG-001: Targets the interaction between β-catenin and CREB-binding protein.
LGK974: Inhibits the secretion of Wnt proteins by targeting porcupine, an enzyme involved in Wnt protein maturation.
Uniqueness of YW1159: YW1159 is unique due to its high potency and selectivity for the Wnt signaling pathway. It has an IC50 value of 1.2 nanomolar in a reporter assay, making it one of the most potent inhibitors available. Additionally, it exhibits minimal cytotoxicity to HEK293 cells, making it a valuable tool for research and potential therapeutic applications .
Biochemical Analysis
Biochemical Properties
YW1159 interacts with the Wnt signaling pathway, a complex network of proteins most well-known for their roles in embryogenesis and cancer . The compound acts as an inhibitor of this pathway, with an IC50 value of 1.2 nM in a reporter assay . This suggests that YW1159 has a high affinity for its target biomolecules within the Wnt signaling pathway.
Cellular Effects
In cellular contexts, YW1159 has been shown to influence cell function significantly. It is not cytotoxic to HEK293 cells following incubations of up to 24 hours, but cell viability is decreased in a concentration-dependent manner after 72 hours . This suggests that YW1159 may have time- and dose-dependent effects on cell viability.
Molecular Mechanism
The molecular mechanism of YW1159 involves its interaction with the Wnt signaling pathway. By acting as an inhibitor, YW1159 can prevent the normal functioning of this pathway, potentially leading to altered cell growth, differentiation, and migration
Temporal Effects in Laboratory Settings
The effects of YW1159 have been observed over time in laboratory settings. As mentioned, YW1159 is not cytotoxic to HEK293 cells up to 24 hours of incubation, but longer exposure times lead to decreased cell viability in a concentration-dependent manner . This suggests that YW1159 may have both immediate and long-term effects on cellular function.
Metabolic Pathways
Given its role as a Wnt signaling inhibitor, it is likely that YW1159 interacts with enzymes and cofactors within this pathway
Subcellular Localization
Given its role as a Wnt signaling inhibitor, it is likely that YW1159 localizes to areas of the cell where this pathway is active
Preparation Methods
Synthetic Routes and Reaction Conditions: The synthesis of YW1159 involves multiple steps, starting with the preparation of the triazole ring. The key steps include:
- Formation of the triazole ring through a cycloaddition reaction between an azide and an alkyne.
- Introduction of the fluorophenyl and quinolinyl groups through nucleophilic substitution reactions.
- Final coupling of the triazole derivative with a carboxamide group under specific reaction conditions.
Industrial Production Methods: Industrial production of YW1159 follows similar synthetic routes but on a larger scale. The process involves:
- Optimization of reaction conditions to ensure high yield and purity.
- Use of industrial-grade reagents and solvents.
- Implementation of purification techniques such as recrystallization and chromatography to obtain the final product with a purity of ≥98% .
Chemical Reactions Analysis
Types of Reactions: YW1159 undergoes various chemical reactions, including:
Oxidation: The compound can be oxidized under specific conditions to form oxidized derivatives.
Reduction: Reduction reactions can be performed to modify the functional groups present in the compound.
Substitution: Nucleophilic and electrophilic substitution reactions can be carried out to introduce different substituents.
Common Reagents and Conditions:
Oxidation: Common oxidizing agents include potassium permanganate and hydrogen peroxide.
Reduction: Reducing agents such as sodium borohydride and lithium aluminum hydride are used.
Substitution: Reagents like halogens, alkyl halides, and nucleophiles are employed under controlled conditions.
Major Products: The major products formed from these reactions depend on the specific reagents and conditions used. For example, oxidation may yield quinolinyl derivatives, while substitution reactions can introduce various functional groups .
Properties
IUPAC Name |
1-(2-fluorophenyl)-5-methyl-N-quinolin-2-yltriazole-4-carboxamide | |
---|---|---|
Source | PubChem | |
URL | https://pubchem.ncbi.nlm.nih.gov | |
Description | Data deposited in or computed by PubChem | |
InChI |
InChI=1S/C19H14FN5O/c1-12-18(23-24-25(12)16-9-5-3-7-14(16)20)19(26)22-17-11-10-13-6-2-4-8-15(13)21-17/h2-11H,1H3,(H,21,22,26) | |
Source | PubChem | |
URL | https://pubchem.ncbi.nlm.nih.gov | |
Description | Data deposited in or computed by PubChem | |
InChI Key |
PNGUNLPOXKPCPK-UHFFFAOYSA-N | |
Source | PubChem | |
URL | https://pubchem.ncbi.nlm.nih.gov | |
Description | Data deposited in or computed by PubChem | |
Canonical SMILES |
CC1=C(N=NN1C2=CC=CC=C2F)C(=O)NC3=NC4=CC=CC=C4C=C3 | |
Source | PubChem | |
URL | https://pubchem.ncbi.nlm.nih.gov | |
Description | Data deposited in or computed by PubChem | |
Molecular Formula |
C19H14FN5O | |
Source | PubChem | |
URL | https://pubchem.ncbi.nlm.nih.gov | |
Description | Data deposited in or computed by PubChem | |
Molecular Weight |
347.3 g/mol | |
Source | PubChem | |
URL | https://pubchem.ncbi.nlm.nih.gov | |
Description | Data deposited in or computed by PubChem | |
Retrosynthesis Analysis
AI-Powered Synthesis Planning: Our tool employs the Template_relevance Pistachio, Template_relevance Bkms_metabolic, Template_relevance Pistachio_ringbreaker, Template_relevance Reaxys, Template_relevance Reaxys_biocatalysis model, leveraging a vast database of chemical reactions to predict feasible synthetic routes.
One-Step Synthesis Focus: Specifically designed for one-step synthesis, it provides concise and direct routes for your target compounds, streamlining the synthesis process.
Accurate Predictions: Utilizing the extensive PISTACHIO, BKMS_METABOLIC, PISTACHIO_RINGBREAKER, REAXYS, REAXYS_BIOCATALYSIS database, our tool offers high-accuracy predictions, reflecting the latest in chemical research and data.
Strategy Settings
Precursor scoring | Relevance Heuristic |
---|---|
Min. plausibility | 0.01 |
Model | Template_relevance |
Template Set | Pistachio/Bkms_metabolic/Pistachio_ringbreaker/Reaxys/Reaxys_biocatalysis |
Top-N result to add to graph | 6 |
Feasible Synthetic Routes
Disclaimer and Information on In-Vitro Research Products
Please be aware that all articles and product information presented on BenchChem are intended solely for informational purposes. The products available for purchase on BenchChem are specifically designed for in-vitro studies, which are conducted outside of living organisms. In-vitro studies, derived from the Latin term "in glass," involve experiments performed in controlled laboratory settings using cells or tissues. It is important to note that these products are not categorized as medicines or drugs, and they have not received approval from the FDA for the prevention, treatment, or cure of any medical condition, ailment, or disease. We must emphasize that any form of bodily introduction of these products into humans or animals is strictly prohibited by law. It is essential to adhere to these guidelines to ensure compliance with legal and ethical standards in research and experimentation.